Origins of Aurora
​
The mid-21st century saw the development of the first space-based solar power systems. They were extremely efficient—22 hours a day of sunlight unobscured by atmosphere and weather—and virtually eliminated the environmental issues related to energy production. They were built from materials mined from asteroids, and required an army of engineers stationed in orbit for months at a time. Housing them required large space stations that could simulate gravity (link).
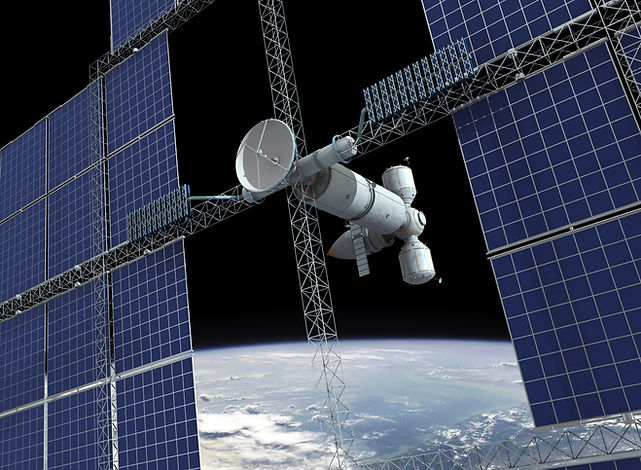
Origins of Aurora
​
The following is adapted from excerpts of Primordial, which takes place in 2280. Portions have been redacted for spoilers. Use the buttons below to toggle spoiler setting:
​
⦿ Spoilers redacted
​
The mid-21st century saw the development of the first space-based solar power systems. They were extremely efficient—22 hours a day of sunlight unobscured by atmosphere and weather—and virtually eliminated the environmental issues related to energy production. They were built from materials mined from asteroids, and required an army of engineers stationed in orbit for months at a time. Housing them required large space stations that could simulate gravity.
​
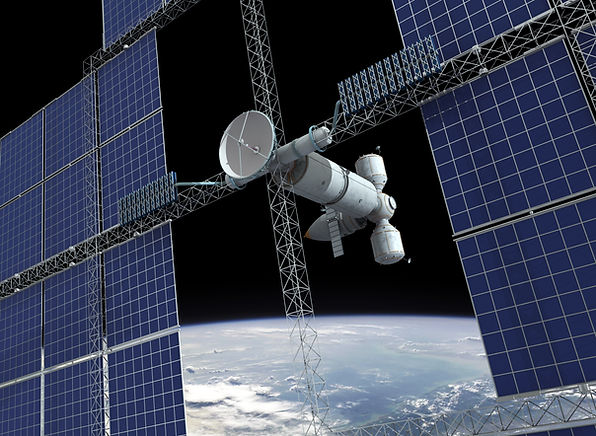
A space-based solar power array in Earth orbit.
Soon virtually all of the world’s electricity and a rapidly increasing share of metals and other minerals came from space and were controlled by one of four major powers: China, India, the European Union, and the Power and Mineral Extraction Alliance, or Pamex. Other countries pretty much had to align with one of them to secure the resources they needed to participate in the world’s economy.
Pamex evolved as an extension of the Five Eyes, an intelligence-gathering consortium of the US, UK, Canada, Australia, and New Zealand.

Offices of the UK’s Government Communications Headquarters (GCHQ), one of the intelligence agencies that is part of Five Eyes.
It quickly grew to include Japan, Brazil, Mexico, South Korea, South Africa, and a number of smaller countries.
​
Governed by a council representing its member nations, it was designed to be self-supporting by selling electricity and minerals. It controlled ships, power-generating facilities, mining operations, and the infrastructure to support them, including the space stations. It turned out to be, not just self-supporting, but enormously profitable, and evolved into an independent economic powerhouse.
​
All this activity in space required a large law enforcement and defense footprint, and Pamex understood from the beginning that a UN- or NATO-style command structure would be inadequate, especially since competitors India and China didn’t need international parleys to make decisions. So Pamex created a largely independent police and military force, which grew in size and power to match the increasing importance of space operations.
Pamex took the lead in developing fusion propulsion and by the end of the 21st century was building ships that could achieve almost one-sixth light speed.
​
In 2098 Pamex launched interstellar probes to seven of the closest star systems.
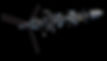
The Emerson, one of seven unmanned, fusion-powered, interstellar probes launched by Pamex in 2098.
This was controversial at the time—some considered it a waste of funds, and public interest was muted by the fact that the first results from Alpha Centauri would take nearly three decades to get back to Earth, and those from the farthest probe, to Tau Ceti, would take 70 years.
The probes were fully autonomous with sophisticated decision-making IA (intelligent agent) software, and equipped with “baby” probes and an array of tools for exploring the kinds of hostile environments that had been encountered on planets and moons throughout the Sol system.
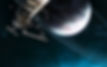
A "baby" probe dispatched by a larger interstellar probe.​
It took the Barnard System probes 31 years to get there and six more years for their reports to reach Earth. After several months of exploring, one of the probes made a surprising discovery: small quantities of statheros on the shores of the methane seas of Pogo.
​
Statheros, commonly called stath, is element 126, and more specifically, isotope st354. It was first created in particle accelerators but never in more than microscopic quantities. The deposits on Pogo were the first natural source ever discovered.
Thanks to its extreme density, stath is capable of deflecting gamma rays while absorbing almost no heat, which would make it ideal for shielding fusion engines if it could be produced in quantity. A single kilogram of stath could replace 200 kilograms of tungsten and reduce the “dry mass” (a ship’s mass with everything but fuel) of a typical ship by half or more. A warship with stath shields would be able to accelerate twice as fast as one without; a freighter would be able to carry two-thirds more cargo. Ships without stath would be obsolete.
Pamex military scientists thought the small deposits of stath on Pogo’s shores might be indicative of large quantities beneath the seas, but to look for this they had to provide revised instructions to the Barnard probes to divert attention from the rest of the star system and focus on this one difficult task—surveying all the liquid methane seabeds mining on Pogo to estimate the quantity of available stath. It would take 12 more years to get results back from this deeper exploration.
In the meantime Pamex took extraordinary measures to keep the discovery of stath secret. The number of people in the know was probably fewer than 30. If it turned out there were indeed large quantities of stath on Pogo, and Pamex could get a significant head start on mining it, they might be able to take control of Pogo and monopolize stath, which would give Pamex hegemony over space travel and all that entailed.
It was immediately understood that mining stath on Pogo would be a monumental challenge, mainly because of the distance from Earth—six light-years. Even with the fastest fusion-engine ships, a round trip to Pogo, including deceleration, would take more than 60 years.
Despite the existence of sophisticated automated mining systems, it was not feasible for a robotic mining operation with a six-year communication lag to self-maintain for more than a year or two. People would be required on-site, people who could not realistically expect to come home. Ever. The long-term feasibility of such an endeavor would require, not just an outpost, but a complete society.
So Pamex started taking small steps—mostly R&D—to prepare for a possible stath-mining colony. If realized, it would be humanity’s first interstellar settlement.
All the R&D had to be dual-use so even the people doing the work didn’t know it had anything to do with stath. Pamex invested in the development of more autonomous machines for mining asteroids and manufacturing colony tubes, and increased funding for academic research into the sociological, economic, and governing requirements for establishing stable colonies in other star systems.
In 2139—about a decade after Pamex learned of the presence of stath on Pogo—the first data started reaching Earth from the probes that had gone to the star system Wolf 359. For the first several months, all the data that trickled in was relatively unremarkable. Then one probe’s optical scanner picked up an anomalous object—an asteroid with a very high albedo, meaning it was highly reflective, unlike anything in the Sol System. Early images were just a few pixels and indicated an object about 120 meters across. If it hadn’t happened to be relatively close, the probe would have missed it.
​
The probe’s IA decided this was worth investigating and proceeded to adjust its orbit to get a closer look. None of this generated much attention on Earth—just another interesting-looking space rock.
As the probe got closer over the next few weeks and the data became more detailed, it gradually became clear that the albedo was too high to be explained by either a layer of ice or a high concentration of metals in the rock. It was almost like polished metal. It also appeared to be quite spherical, which would be very odd for an asteroid this small; asteroids needed to be over a thousand times bigger to have the gravity necessary to overcome their internal resistance and form a sphere (which is the reason planets and most moons are spheres).
The story was now attracting a lot more attention. Government and university scientists offered possible natural explanations. The popular press, picking up on the fact that astronomers were having a hard time explaining it, sought out technical people—typically those without a professional reputation to worry about—who were willing to speculate publicly about possible extraterrestrial origins.
As the probe got even closer, it became clear the object actually was an almost perfect sphere with a shiny surface. It was now the subject of daily headlines. Eventually the probe pulled up in an orbit less than a hundred kilometers away and the photos left no doubt.
The sphere, which was spinning slowly, had a smooth surface but with hundreds of dimples of various sizes, sort of like an odd-looking golf ball. It also had what appeared to be scarring—streaks on the shiny surface—presumably from random glancing collisions with space rocks. Otherwise the surface was unblemished and unbroken. There were no openings or protrusions, nothing that looked like hatches, nozzles, or sensors.
In the academic community, while this discovery was obviously surprising, it wasn’t shocking. Scientists had long believed that extraterrestrial life was likely. It was a simple matter of statistics. With a trillion planets in the Milky Way alone, there might be more than ten billion with conditions conducive to the emergence of life. With such huge numbers, it was reasonable to think a tiny percentage might actually have led to some form of life, and that a tiny percentage of those life forms might have evolved into spacefaring civilizations. In addition, the universe had been around for more than 13 billion years, while Earth had been exploring space for less than 300. If just one spacefaring civilization came into existence every hundred thousand years on one of those trillion planets, there would have been 130,000 of them in the history of the Milky Way. And spacefaring civilizations might be expected to send out probes to other star systems, just as Earth had.
So before launching its probes, Pamex military had to decide what the probes would do if they came across signs of an extraterrestrial civilization. This was a question with potentially existential ramifications for humanity because there was no way to know whether any civilization they found would be friendly or hostile. In fact, there was a whole body of work making the case that a civilization would be more likely to be hostile. Humans had been interstellar for less than a hundred years; any other interstellar race was likely to be hundreds, thousands, even millions of years more advanced. If they encountered humans, assuming their primary concern was their own survival, their safest course might be to wipe us out before we could advance technologically and become a threat. Even if one disagreed with this logic and thought there was a high probability they would be friendly, it just wasn’t worth the risk of total annihilation.
Given this risk, it was prudent for humans, when exploring, to do so stealthily. In fact, this logic is one possible answer to the famous Fermi Paradox, which poses a question: with billions of habitable planets and billions of years to evolve, there should be plenty of spacefaring civilizations out there, so why haven’t we seen them? The answer, perhaps, was that other civilizations were also being stealthy so as not to draw the attention of more advanced ones. An alternative answer was that one or a few super-advanced civilizations had wiped out all the others, and Earth just hadn’t come to their attention yet.
One option the military planners had considered was that if the probes discovered something, they could simply stay away and even go silent. On the other hand, passing up the opportunity to gather intel on a potential threat carried its own risks.
Instead, it was decided the probes would gather intel, but discreetly, in a way that disclosed as little as possible about themselves. They wouldn’t make physical contact or use directed radiation, as they normally would when exploring a moon or asteroid. They would examine from a distance, using only passive sensors.
When the Wolf 359 probe encountered the presumably extraterrestrial orb, it used visual and hyperspectral imaging, gravimetric analysis, neutrino transmetrics, and passive spectrometry based on radiation reflected off the orb from external sources. It was determined that the surface was composed of an unknown alloy of titanium, nickel, chromium, and traces of other metals woven with carbon. The orb was apparently not creating any energy internally, either thermal or vibrational, and didn’t seem to be communicating or adjusting its orbit. Based on a statistical analysis of the impact scarring, scientists estimated it had been in orbit for 450 million years, give or take a hundred million.
Given its age and apparent inertness, it came to be popularly known as the Relic, despite the fact that scientists warned there was no reason to be sure it wasn’t just in a dormant state, and that the age didn’t mean the civilization that had built it wasn’t still around. Indeed, although scientists didn’t know if or how it propelled itself, it was possible it could have arrived in the system from somewhere else just recently, and could even head elsewhere at any time.
There was widespread speculation about what function the Relic served; it was tempting to assume it was some kind of probe, but there really wasn’t any evidence one way or another.
Gravitational analysis determined the total mass, from which the overall density was calculated as roughly 4,000 kilograms per cubic meter, which was in between that of aluminum and titanium. The grav and neutrino sensors were expected to yield information about the internal structure, but the data they collected was completely undifferentiated. This meant that either the orb was uniformly solid, which seemed unlikely, or the gravitational and neutrino signals were somehow being blocked, which would imply the Relic had technology or materials far more advanced than anything on Earth.
Decisions about the probes’ original programming had been made decades ago when no one thought an encounter with aliens was likely. But now those decisions were being relitigated intensely and on a global scale. Many people wanted Pamex to send the probe new instructions to investigate more aggressively, arguing we should learn as much as we could about what was quite possibly the most significant discovery in history. At the other extreme, India and China proposed that Pamex be internationally banned from investigating further so as not to put humanity at further risk—which conveniently aligned with their interest in preventing Pamex from discovering any advanced alien technology that it could keep for itself.
Ultimately Pamex leadership stuck with its original reasoning that more aggressive measures would be too risky. The probe continued to monitor the Relic, but only passively.
While all this was going on with the Relic at Wolf 359, data from the Barnard probes, which had been sent instructions to focus on how much stath was on Pogo, started to come in. By the beginning of 2141 it was clear there was a lot of stath on Pogo, readily available in nodules on the seabeds, with more buried underneath.
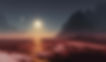
A view from the surface of Pogo showing stath nodules on the shore of one of its liquid methane seas, with Moira and Barnard's Star in the background.
Still only a very small group of people in Pamex knew about the stath. The development of a mining colony would eventually require large public appropriations, at which point the whole endeavor would have to be disclosed, but they hoped to maintain secrecy for as long as possible to extend their head start on their adversaries. By shifting some existing budget allocations and securing additional black funding, they now significantly stepped up their planning and R&D.
The bigger the program got, the harder it was to keep secret. Soon there was speculation in the Western press about it and indications that foreign intelligence services were poking around. Then an opportunity presented itself.
A year after the confirmation of stath and two years after the discovery of the Wolf Relic, a probe in the Lalande system reported spotting an asteroid with extremely high albedo. The probe (which, due to the distances involved, knew nothing of the Wolf Relic) investigated. The object turned out to be a giant metallic golf ball, identical to the first in most respects. It was the same size, had the same kind of dimpling, and appeared to be roughly the same age, 450 million years old. It also showed no signs of activity, and passive sensors again failed to reveal anything about its interior.
There were two differences. First, while the dimples on Relic-2 were roughly the same in size and number as those on Relic-1, they were in a different pattern, implying some unknown difference in function. And second, whereas Relic-1’s orbit was significantly elliptical and 20 degrees off the ecliptic, Relic-2’s orbit was nearly circular and on the ecliptic.
Typically, random gravitational interactions with passing asteroids or planetary alignments would eventually disturb the orbits of small bodies like this. Relic-2’s seemingly undisturbed orbit might imply it had arrived in this system relatively recently, sometime in the last million years or so. Alternatively, it might have been doing station-keeping—maintaining its orbit using hidden thrusters or some more exotic means of propulsion. Or it was possible the circular orbit was just a matter of natural chance.
The two Relics’ proximity to Earth’s solar system didn’t mean the alien civilization was in the immediate vicinity. The fact that they were in two nearby star systems might be a coincidence—450 million years ago the two stars would have been much farther away from each other and from Earth. In addition, for all anyone knew, the civilization that had built these two might have sent out thousands or even millions of them. In fact, this raised the disturbing possibility that one could be orbiting undetected in the far reaches of Earth’s Solar System, although scientists deemed this unlikely due to how reflective and thus noticeable they were.
Within six months of the discovery of Relic-2, a series of stories appeared in the mainstream press quoting unnamed sources saying Pamex had set up teams of engineers tasked with investigating a third Relic that had secretly been discovered in the Barnard System. Pamex officials denied it. Follow-up stories claimed the third Relic was in a more “open” condition than the first two and that Pamex thought it could extract technology from it. Pamex issued more denials. Tabloids published outlandish stories that Relic-3 was alive and moving and communicating, which may have contributed to a decline in interest from the mainstream press.
​
A year later China and India formally complained at the United Nations that Pamex was secretly planning to send a mission to BS to take control of Relic-3 and acquire sophisticated technology, a destabilizing act that could also attract the attention of a potentially hostile alien civilization. Pamex was forced to respond in detail, saying they had no evidence of a new Relic in the BS or anywhere else, and so had no plans for a mission to retrieve it. But the complaint revived interest from the Western press, which uncovered details about specific Pamex programs that seemed to be geared toward an interstellar colony mission. The response from Pamex was that these programs had nothing to do with Relics, but that Pamex was seriously interested in the possibility of establishing colonies in other star systems at some time in the future, an ambition that enjoyed broad popular support.
Pamex was governed by a council made up of representatives of the member countries, but until now the stath secret was known only to a small management group plus the Select Intelligence Committee of the Council. By 2155—seven years after the confirmation of plentiful stath—they’d reached the point where they had to start building ships and recruiting and training colonists, which could not be done in secret. They publicly released a comprehensive budget for the stath-mining colony and presented it to the full Council.
Their plan required a huge investment, which would be financed with long-term bonds, and which would be recouped from the stath profits. They made the case that Pamex had paid 100% of the cost of the probes that discovered stath, and thus deserved to control it. The plan met with high levels of support from Pamex’s member nations.
The other three space powers weren’t happy. China was particularly belligerent, accusing Pamex of deceiving the whole world and saying it considered this a hostile act. It declared that it could never allow Pamex to have exclusive control of stath.
After a period of escalating diplomatic tensions and fleet maneuvers, China attacked Pamex’s lunar shipyard, where work had already begun on the colony fleet. Pamex struck back, and the conflict spiraled into the first war between two major powers in over a century, as well as the first significant space combat in history. The Shipyard War ultimately resulted in more than 12,000 fatalities, including civilians, and massive damage to ships and facilities.
The war ended with the Treaty of Bangalore, which established rules for the colonization of the Barnard System and the mining of stath on Pogo.
The Western press, and later historians, dissected the origins of the Relic-3 story, but never settled who the sources were and why they were considered credible. Nor was it ever determined with certainty what caused China and India to reopen the controversy a year after the original story had fizzled out. Some people clung to the belief that there really was a Relic-3. Others attributed the whole thing to sloppy reporting and derisively called it the case of the AWOL Aliens. But the most popular theory was that it was an elaborate misdirection: Pamex had planted the original story and took further, clandestine steps to convince China and India it was true, all to preserve the stath secret. The popularity of this theory was cemented by a star-studded movie in the 2160s called The Emerson Deception. (The probe that went to the Barnard System was named the Emerson after the astronomer Edward Emerson Barnard.)

A poster for the blockbuster 2161 production of The Emerson Deception.
If it really was a misdirection, it apparently worked: the other powers must have believed that Pamex’s preparations really were aimed at investigating Relic-3, and decided that investigating it themselves wasn’t worth the expense. By the time the stath discovery was made public, they were almost a decade behind Pamex in planning and developing a colony for the Barnard System.
However, if Pamex’s goal had been to monopolize stath, they failed. They’d hoped to get such a big head start that their adversaries would deem it too costly to mount a military challenge to Pamex’s control of Pogo and stath. But Pamex had underestimated the extent to which the Chinese would view stath as an essential strategic asset—and thus underestimated their willingness to go to war over it.
In the years after the Shipyard War, the four space powers agreed to a treaty that addressed interactions with the Relics and any other alien technologies encountered in the future. Acknowledging the risk to all of humanity, it forbade any vessel from approaching closer than 1,000 kilometers, prohibited the use of all but passive, non-emissive sensors, and required that any future encounters be disclosed publicly and promptly.
The treaty also required that any further missions to the two Relics be conducted jointly. Over the next 50 years, two more sets of probes were launched. Thanks to more advanced passive sensors, they were eventually able to determine that the 120-meter-diameter Relics had a 10-meter-thick outer shell with a density slightly higher than that of lead, surrounding an inner sphere with a density 1.5 times that of water. Interestingly, the sensors indicated that, accounting for the thermal gradient, there was a high probability that the composition of the inner sphere was uniform down to a scale of microns and possibly even nanometers. In other words, the interior was apparently a uniform mass, though whether it was solid, liquid, or gelatinous could not be determined.
Ultimately, while speculation abounded, the probes never uncovered enough information to draw conclusions about the Relics’ origin or function, or even to determine whether they were truly relics or still somehow active.
Pamex’s colony ship departed the lunar shipyard in 2163 and arrived in the Barnard System 34 years later to establish humanity’s first interstellar settlement, Aurora. China and India followed with their own stath-mining colonies, with Hongzin founded nine years after Aurora, and IMEC (the Indian Mining Enterprise Consortium) three years after that.
From the outset, relations between Aurora and Hongzin have oscillated from tense to overtly hostile. Over the years there have been several skirmishes that threatened to spill over into all-out war.
On December 7, 2280, an autonomous shuttle launches from Aurora with three people on board. When it lands in Hongzin, it’s empty, with no indication where the passengers went.
​
History of O'Neill Cylinders​
By the late 2040s it was widely understood that humans could not thrive in space without Earth-like (1g) gravity. Living for long periods on the moon (.18g) or Mars (.38g) wreaked havoc on various body systems. Time-consuming exercise and drugs could only partially ameliorate the problem and, in any case, were not a good long-term solution.
A spinning structure can simulate gravity, but the concept was difficult to test because there was no way to build a useful simulator on Earth. It had to be tested in space, and this was first done in the 2040s, with small habitats attached by lightweight girders to a counterweight.
Next came the development of small experimental toruses, like the one shown below.

A small experimental rotating torus habitat retrofitted to an existing space station
However, the torus configuration is inefficient—usable living area is limited to the inside surface of the outer part of the ring.
The optimal shape for a colony is a cylinder. This has been known since the 1950s. It was first suggested in science fiction novels by Oberth (1954), Niven (1970), and Clarke (1973).
Princeton professor Gerard K. O’Neill published a more rigorous study of the concept in 1976. Though he called the idea “Island 3,” the design has come to be called the O’Neill cylinder, although O’Neill’s specific design was never actually built.
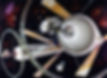
NASA artist’s depiction of O’Neill’s cylinders.
Construction of the first small rotating cylinder colony began in 2070 in Earth orbit and was completed in 2076. Clarke Station consisted of two tubes, each 1,000 meters in diameter 3,000 meters long, that spun at 1.1 revolutions per minute and produced 0.67 g. It housed 25,000 people employed in asteroid mining and space-based solar power. In 2090, the 2-km-diameter, 6-km-long Katinawa Station was built in lunar orbit. And in 2122, construction began on the 4-km-diameter, 12-km-long O’Mara Station in Mars orbit. This was the first one built almost entirely from locally mined asteroids and, due to the distance from Earth, designed to be fully self-supporting.
Shortly after the discovery of stath on Pogo in the Barnard System in 2136—a discovery that Pamex went to great lengths to keep secret—Pamex began designing the largest space station yet. Called Vanica, it consisted of twin 8 x 22-km tubes to be built in the densest part of the inner band of Sol’s asteroid belt, 2.34 AU from the sun—1/3 of the way from Mars to Jupiter. One of Vanica’s purposes—unbeknownst to anyone but a top-secret committee within Pamex—was to serve as a prototype for a future interstellar settlement that would mine stath in the Barnard System. The lessons learned from Vanica were applied to the design, construction, and operation of Aurora.
Differences between the O'Neill and Aurora Designs
​
There are four obvious differences between O’Neill’s classic Island 3 design and the Aurora colony:
​
1) Windows and Mirrors
​
In O’Neill’s design, each tube is divided lengthwise into six strips, three of which are transparent windows (see images below). Outside of each window is a long, hinged mirror designed to track the sun and reflect its light into the tube. But this design is problematic for multiple reasons: a) it wastes half the “land” area; b) there are large mechanical stresses on the rotating mirror structures; c) the windows make poor radiation shields; and d) they provide generally unappealing views of a constantly rotating space environment. In addition, in the Barnard System, they would do little to generate light or power due to the distance from the star and its inherently low output (less than 4% of Sol’s total radiation output).
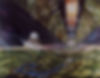
An artist’s concept of the interior of an O’Neill cylinder, showing the three long windows.
2) Configuration
​
In both O’Neill’s design and Aurora's, the tubes counter-rotate (although not for the reasons many suppose). But in O’Neill’s design, the tubes are oriented side by side to maximize their mirrors’ exposure to the sun. The Aurora tubes, on the other hand, since they don’t need to point toward the star, are oriented end to end, which simplifies their physical connection and movement between the two tubes (although transitioning between tubes is still complex).
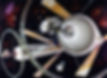
O’Neill’s configuration featured two cylinders side by side.
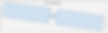
Aurora’s two cylinders are aligned end to end, with a takeoff and landing platform in between.
3) Platform
​
In between Aurora's two tubes is an expansive platform, stationary relative to the tubes, that serves as a takeoff and landing deck for ships and as a bridge between the two tubes for pedestrians and hoppers. Anything leaving one of the tubes must first “spin down” to transition from the rotating tube to the stationary platform, a complex process.
​
​
4) Pods vs. Terraces
​
In O’Neill’s design, agriculture takes place in small, separately rotating pods arranged in a circle outside the tubes, each of which has its own gravity (based on rotational speed) and environment to optimize conditions for different crops. While the pods look small in the image below, each is actually 3.2 km in diameter and has quite a bit of land area. But this design would create numerous logistical issues: each pod requires separate infrastructure for water, air handling, shielding, power, and control systems. In addition, transportation of supplies, people, and product to and from the tubes would be time consuming and complex. In the Aurora design, agriculture takes place on huge terraces at the “south cap.” Gravity for each terrace depends on its distance from the center. Lighting, and to a lesser extent humidity, can be varied from area to area.

O’Neill’s configuration: agricultural pods in a ring outside the cylinders.
Colony features ​
​
Size
​
Each of Aurora’s two tubes is 23 kilometers long and 8 km in diameter. The “land” area of the two tubes is surprisingly large—over 4,500 square kilometers, including agricultural space on terraces and industrial space inside the caps.
Aurora’s two tubes are designed to accommodate a population of 400,000. By comparison, the whole city of New York is approximately 780 square kilometers. Considering NYC’s population is in the millions, Aurora’s 4,500 square kilometers may seem excessive. However, sustaining a population requires much more than just living areas. People need power, food, manufacturing, transportation, and recreation, all of which require additional space. NYC’s land area doesn’t include the farms from which people get their food, nor most of the factories from which they get their products.
With a current population of 90,000 in 2280, things are quite spacious—just 20 people per km2. Even at the projected population of 400,000 in another 40 years, the density will be 88 people per km2. By comparison, the contiguous US is 43, and France is 117.

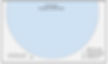
Layout​
In between the tube’s exterior and “ground” level, there are three “underground” levels, each roughly 10 meters high:
-
the sublevel, used primarily for deliveries, garbage collection, and other services
-
the infrastructure level
In areas away from skywire bases, the ground level varies in elevation by as much as 20 meters, allowing for natural-looking, undulating terrain. Rivers and lakes of various sizes are built into the terrain, some as deep as 15 meters but most less than 3 meters.
The tube has structural limits on how much weight it can support, and all construction requires detailed load analyses. Generally, to stay within comfortable safety margins, ground-level construction is limited to relatively sparse concentrations of 2-story structures, mostly homes and small commercial buildings.
Where there are skywires to provide additional structural support, there can be mountains as tall as 300 meters (with aerogel filler beneath the surface) or buildings up to 20 stories tall.
Much of the industrial activity—notably mining, refining, and a great deal of fabricating—takes place off-colony around the asteroids. Almost all other industrial activity takes place in the caps or under the lowest terrace. Some processes have been optimized for reduced gravity and take advantage of the upper levels of the caps or in the Axle.
​
​
Axle
​
Each tube has an Axle, a 200-meter-diameter “pipe” that runs through the center of the tube from one cap to the other. From the ground, if you hold your hand at arm’s length, it’s about the width of two fingers, but up close, it’s a massive structure.
​
The Axle serves four main purposes: 1) longitudinal spin rigidity, 2) light, 3) rain, and 4) access to weightlessness for manufacturing and entertainment.
​
1) Longitudinal spin rigidity: The Axle is effectively a taut cable that helps keep the tubes and the two caps aligned when the orientation of the structure is adjusted (for example, during a Random Positional Change).
2) Light: The outer shell of the Axle includes light-emitting panels designed to simulate daytime on Earth. Circadian rhythms of flora and fauna, including humans, depend not just on day and night but on the position of the sun in the sky. The lights on the Axle include a brilliantly lit bright spot that moves from one cap to the other during the day to match the Earth's sun. The lights also turn on gradually at dawn and turn off gradually at dusk, and change colors during these times to simulate what real sunrises and sunsets look like.
3) Rain: The Axle produces a light shower every day at 5:30 a.m. for 30 minutes to provide watering for plants and to rinse off surfaces. In addition, a few times per month, the colony schedules rains at certain times during the day and night, as most people prefer some variation in the weather. (Because the climate in the colony is controlled, it could be “nice” every day if that's what people wanted.)
4) Manufacturing and entertainment: Most zero-g manufacturing takes place in space outside the tubes, but there is also some space for it in the Axles. Entertainment facilities include ultra low-g swimming pools, zero-g gyms, and courts for bolo, a sport similar in some ways to water-less water polo but played in zero-g. Aficionados on Aurora consider bolo more entertaining than any terrestrial sport due to the complex swim-bounce moves the players must make.
There is a small pedestrian beltway for people to get around inside the Axle, specially designed for weightlessness, and a 20-meter-wide “highway” for hoppers and emergency vehicles.
​
​
Skywires
​
A skywire is a 20-meter-diameter column that spans the 8-km diameter of the tube. Aurora has 19 of them spaced out along the length of the tube. Each one is offset 18 degrees around the circumference, meaning they are arranged like the rungs of a double helix. At the center of each skywire are 13 cables, each 2 meters wide and consisting of tens of thousands of twisted carbon nano-fiber filaments.
A common misconception among young Auroran children is that skywires hold up the other side of the tube, keeping it from crashing down on people’s heads; in fact the opposite is true: the skywires help hold the tube together, keeping it from being flung apart by the centripetal forces of the rotation.
The tubes are reinforced at the 38 bases of the 19 skywires and can support more weight there, so skywire bases can be surrounded by high-rises of up to 20 stories or hills as tall as 300 meters (hills can be taller than buildings because their solid-rock exteriors are filled with low-density aerogel).
Each skywire also contains a dual elevator system for pedestrians. One type of elevator is for transportation from the ground to the Axle. The other type is an express elevator that does not stop at the Axle but rather goes straight through to the opposite side of the tube. The 8-km trip takes about 5 minutes including waiting time, which is substantially less than the roughly 20 minutes it would take on the belts along the circumference of the tube.
​
​
Caps
​
At the ends of the tubes there are fully enclosed megastructures called the caps. South Cap on A Tube was the first part of the colony constructed, and housed the Originals for several months after they arrived.
The interior faces of the north and south cap of each tube look very different: the north one is a huge mirror, while the south one has the terraces attached to it, and only the center of it is mirrored. When you’re looking north, the North Cap mirror makes it look like the tube extends another 23 kilometers.
​
A note on directions: The assignment of north, south, east, and west was arbitrary and had to be consciously decided by the designers. The cap closest to the platform is called South Cap in both tubes. The configuration of both tubes is the same, with terraces on the cap nearest the platform. Although this may seem counterintuitive when you look at the schematic below, it’s actually more intuitive when you’re in the tubes: it doesn’t matter which tube you’re in, the terraces are always south. The alternative would require that when determining directions you’d have to take into account which tube you’re in.
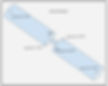
Geometry and Size of the Caps
​
Unlike the rest of the tube, which is open (meaning you can look up and see straight to the other side), the caps consist of concentric levels. There is some variation among the four caps but each one has approximately 150 floors with ceiling heights ranging from 4 meters for standard office space to 120 meters for power plants and hangar decks for mining freighters.
Because each level forms a ring, there is an enormous amount of space. The ground floor alone—the outermost ring—has more than 70 times the square footage of the Pentagon. Altogether, each cap has more than 750 square kilometers of space, which is more than the surface of the interior of each tube, and more than three times the area of Washington DC. (See “Size” for a discussion on population density.)
​
Utilization of the Caps
Most of the space in the caps is taken up by infrastructure and manufacturing facilities, from factories and power plants to air treaters and water pumps.
​
The South Caps of both tubes house the spinners and facilitate the passage of pedestrians and hoppers from one tube to the other, and the transfer of ships to and from the platform where they launch and land.
South Cap on A Tube contains Ascension Station (the colony’s port) and the headquarters of the Ministry of Defense, commonly called Glass Base, which occupies three of the outermost levels along the space-side face of the cap. Glass Base is so named because many of the offices have windows that look out into space.
All four caps can be sealed off in an emergency and be self-sufficient for an extended period, with power, air and water handling, and enough provisions to sustain the inhabitants until the backup hydroponic systems can start producing food. Since the caps had to be fully enclosed and self-sufficient during the construction of the tubes, it only made sense to retain this capability for emergencies. Likewise, either cap on a tube can be closed and the other cap can provide adequate services to replace it for an extended period.
​
Terraces
Each tube has 6 terraces attached to its South Cap devoted mostly to hydroponic agriculture.
The terraces are essentially shelves, attached as concentric rings to the wall of the cap. The lowest, outermost shelf, 400 meters above ground level, extends 3 km out from the cap. It has a diameter of 7,200 meters. The next shelf up extends a little less far, and so on, creating the terraced look. The highest, innermost terrace is 2,400 meters above ground (1,600 meters from the center). The center of the cap wall is 3,200 meters in diameter and is flat and mirrored except for a few openings and landing platforms that jut out. From a distance, the terraces look a bit like a broad, shallow funnel.
The terraces are lightweight, with just enough structure to support the crops, soil, water, lights, and robotic planting and harvesting systems. In addition, g forces decrease closer to the center of the tube, so each terrace, in addition to having less mass than the one below it, also has less gravity weighing it down. The top terrace exerts only 1.5% the weight of the bottom one. The terraces are supported by large cables that run at angles back to the cap wall.
There are lights on the bottom of each terrace to provide light for the plants beneath.
The two terrace systems combined (A Tube and B Tube) have 366 square kilometers of surface area. On Earth, using conventional farming methods, this would have supported about 60,000 people. But hydroponics with vertical growing systems is about 10 times as efficient. In addition, many plants have been optimized for low gravity, and expend less energy on their support structures (e.g., vines), and so are even more productive. The terraces could generate food to support up to 600,000 people comfortably, more than the projected population of 400,000.
The space underneath the lowest terrace is generally used for industrial activity, since the terrace constitutes a “ceiling” that makes it an unappealing place to live (although it is still a very high ceiling—400 meters).
Platform
In the process of designing the first space colonies, an important question was how ships would land at the rotating tubes. There were several options, which can broadly be grouped into a) landing directly in hangars in the tubes, b) docking with ports on the exterior of the tubes, or c) landing on a stationary platform attached to the exterior of the tubes. All have their advantages and disadvantages.
Landing in the tubes would require large openings in the tubes—essentially hangars that are in vacuum. You could put the hangar at the very center of the cap, in which case an incoming ship would just need to “spin” itself to match the rotation of the tube, fly in and land, then be processed through airlocks. The problem is that only one ship could land in the hangar at a time, and this was deemed unacceptable given the large volume of mining traffic that would be required to build and maintain the colony.
If, instead, you put hangars on the cap away from the center or on the exterior curve of the tube, an incoming ship would be presented with a target that was moving in a circle, not spinning. To stay aligned with the hangar opening, the ship would need to apply a constantly changing thrust, a maneuver that would be complex and dangerous and would potentially consume substantial fuel.
​
The second option—external docking ports—suffers from the same drawbacks as direct entry to hangars.
A stationary platform, which is the option the colony designers decided on, is not without issues. One is that maintaining a solid connection between the platform and the tubes, which are themselves not perfectly stable due to inherent asymmetries, presents a significant engineering challenge. But since the two tubes need to be connected anyway, this is less of a problem.
Another issue is that landed ships and/or cargo still need to transition to the rotating tubes. But since passengers and goods need a system to transition from one tube to the other, counter-rotating tube anyway, this is also less of a problem.
The platform is a huge structure, 3,000 x 3,000 meters. To avoid damaging the colony with radiation, approaching ships turn off their fusion engines at least 10 kilometers out and coast slowly in, using retro thrusters for the final approach. Directed by Air Traffic Control (an anachronistic name still in use on the colony), ships land and take off on both sides of the platform (there is no up or down). With no spin and no gravity, the process is simple. Ships are inspected for contraband, weapons, nukes, and pathogens, then towed, either to parking spaces if they are autonomous and do not require servicing, or through airlocks where they make the transition through the spinners and to the hangar deck.
Construction of the Tubes​
​
The Auroran colony ship, the Carifellan, departed Pamex’s lunar shipyards in 2163 with 400 passengers. Six unmanned Avant ships had departed a year before, traveling faster than the larger colony ship. They arrived in 2193, four years before the colonists. Each carried 2,500 tons of robotic mining and manufacturing equipment, spread out amongst them so the loss of a single ship would not by itself have sunk the mission.
The Avant ships had detailed resource inventories compiled by earlier robotic probes, so they could immediately commence mining operations. They worked in the cloud of Trojan asteroids around the L4 Lagrange point of Moira/Pogo, which was where Aurora was to be constructed.
They deployed huge automated machines that engaged in an elaborate process to crush, separate, and purify minerals from asteroids and then alloy them, fabricate parts, and begin constructing the tubes. This complex choreography had been perfected over the previous hundred years of space station construction in the Sol System.
When the Avant ships arrived in the Barnard System, they were six light-years from Earth, but by then, the Carifellan was less than 0.7 light-years away and getting four light-hours closer every day. Thus, the colonists were able to monitor progress and even provide direction with a relatively small and ever-shrinking time lag. When they arrived, there were no major surprises.
As one might expect, the construction process was complex. First to be built was South Cap of A Tube and the attached takeoff-and-landing platform. Once the exterior of South Cap was built, it would be spun up to produce gravity. Next, North Cap would be built, spun up, and connected to South Cap. Then construction would begin on the exterior of the first 7-km length of the tube. While South Cap is 8,060 meters in diameter, North Cap is narrower—7,960 meters. The tube segments are the same diameter as South Cap. This way, they could be constructed around the narrower North Cap. When the first tube segment’s exterior was complete, North Cap would be disconnected from South Cap and then slid along rails to the end of the tube segment. Finally all three sections (the two caps and the tube section) would be sealed together and pressurized.
Some parts of the construction process were meant to be completed by the time the colonists arrived in 2197. The plan was that South Cap and North Cap of A Tube would both be sealed, connected, operational, and habitable; the platform and the ultra wideband Earth communications dish would be operational; and work would have just begun on the skeleton of the first 7-kilometer tube section. The colonists were supposed to move into South Cap while the first tube section was finished.
Unfortunately, when they arrived, things were significantly behind schedule.
South Cap’s exterior had been built, as had the internal portions most easily constructed under zero g. It had then been sealed and spun up to 1g so that the interior and infrastructure systems for power, atmosphere, initial food production, and water circulation could be completed under normal gravity. The platform, which was necessary for efficient ship traffic to and from the now-spinning colony, was also complete.
However, water treatment and waste and recycling systems were not working. As importantly, robotic miners had failed to locate sufficient quantities of phosphorus, which, along with a working water system, was essential for aquaculture and their food supply.
North Cap, which was supposed to have been mostly completed, was at least three months behind schedule and not yet pressurized.
While the faulty waste handling and recycling was a problem, the bigger issue was food. Without water and adequate phosphorus, they had no hydroponics, so they could not generate their food supply. The plan had allowed for some schedule slippage, but the ultimate backup was to refuel the Carifellan from the hydrogen clouds of Moira and make the return trip to Earth using the rest of the food they’d brought with them.
Although they did fill the Carifellan’s fuel tanks in case they decided to abort, they worked on solving the problems. They had four weeks to make a go/no-go decision, after which they would have insufficient food to make the return trip. Starving to death in the Barnard System would be a bad way to fail the mission.
They succeeded in repairing the control software for the robotic assemblers that had messed up the water and recycling systems. They also searched for and found more phosphorus in the asteroids.
They lived for more than six months on rationed food and water in makeshift quarters on South Cap, but in the end they got all systems online.
Like the tubes, the total diameter of South Cap is 8,060 meters. The outer 30 meters holds three service levels, so “ground” level has an 8,000 meter radius. The tube spins at exactly 0.4727 RPM so that at ground level, simulated gravity is an even 1g.
While living in South Cap was not the pleasing, open-space experience that living in the tubes would be, there was plenty of room. The cap is 500 meters long at ground level, so the living area at 1g is 12.5 million square meters. This is about 15 acres per couple. And that doesn't include all the other levels of the cap—unlike the open tube sections, the caps contain several hundred levels, all the way up to the Axle. Each level has a little less gravity than the one below it.
The colonists pressurized North Cap, which could then be spun up and connected to South Cap. At the same time, work proceeded on the external shell of the first tube segment, which was 7 km long. When the shell was complete, North Cap was disconnected from South Cap and then slid along rails to the end of the tube segment—an enormously complex step that required human supervision.
At this point, work could begin on the interior of the new, open tube segment, including its agricultural terraces, Axle, skywires, water features and mountains, and recreation areas.
Rather than working on the next tube section of A Tube, the external mining and construction machines began work on B Tube’s South and North Caps. When these were complete, they would be spun up (in the opposite direction to A) and then connected on the opposite end of the platform.
There was a reason for beginning work on B Tube before finishing A. The spin of A Tube created a gyroscopic effect that made it difficult to adjust the colony’s orientation, which is occasionally necessary to avoid asteroids and to lower the likelihood of a Javelin strike. The counter-rotation of B Tube offsets this gyroscopic effect: although each tube spins, the total system spin (the two tubes combined) is closer to zero.
Today in 2280, the exterior of the northernmost 5-km section of B Tube has just been completed and work has begun on its interior surfaces.
The Belts​
​
Almost no one in Aurora owns a private vehicle. The sublevel—the topmost underground level, just below the surface—serves as a roadway system for the mostly robotic vehicles that provide deliveries, garbage collection, and other services. But the colony planners decided that widespread ownership of private cars carried too many disadvantages, from manufacturing and electricity costs to noise pollution to the space that would be needed for parking lots. They thus designed Aurora to be as walkable as possible.
However, the tubes were large enough that some form of public transit was necessary. From the experience of earlier colonies in the Sol System, the planners knew that Aurorans would probably have little patience for waiting even small amounts of time for a bus or subway, and would often resort to ordering hopper taxis, an inefficient alternative.
So instead of buses or subways, Aurora uses a system that was introduced on Vanica Station and proved popular there: a network of moving walkways called “the belts.”
The belts are housed one level below the sublevel. There are four “north/south” mainline beltways that run straight from one endcap of the tube to the other. They are a little more than 6 km apart from each other. Each mainline consists of two 15-lane “streets”—one northbound, one southbound—except each lane is a moving walkway, called a belt. There is a platform, like that in a subway or train station, between these two streets.
The belt closest to the platform is the slowest and moves at 3.2 kilometers per hour, the speed of a slow walk. To get on, you walk on the platform alongside this belt and then step onto it. As you continue walking, you can then step onto the next belt over, which is moving a little faster, 6.5 km/h, and then onto the next at 9.7 km/h, and so on. The last lane, the 15th, is the fastest at 48.6 km/h. By walking at a normal pace of 4.7 km/h, you can increase your speed to 53.3 km/h, or about 33 miles per hour. It sounds dangerous but the belts next to you always have a very low relative velocity. To exit the beltway, you reverse the process, stepping onto progressively slower lanes until you reach the platform.
If you go down one level lower, you can access the six “east/west” beltways that run along the circumference of the tube. There is one inside the base of each cap, and three between them, spaced 5 km apart. They work the same as the N/S mainlines, with 15 lanes in each direction.
In between the mainlines every 250 meters are feeder belts, with just six lanes each direction, which max out at 19.4 km/h. On the surface, you are never more than 125 meters from a feeder or mainline belt.
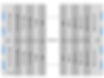
Diagram of a feeder belt station. The speed of the two innermost, slowest lanes—3.2 km/h—is the speed of a slow walk. Mainline beltways have 15 lanes per direction, with the fastest going almost 50 km/h (30 miles per hour). On both feeders and mainlines, there is seating along the outer edge of each outermost, fastest belt.
There are several significant advantages that the belts have over more traditional public transit systems. With buses and subways, no matter how frequent they are, you’ll always experience some waits, often substantial ones. If you want to try to minimize waits, you have to track schedules. By contrast, the belts run continuously. This also means they never stop to let people on and off the way buses and subways have to, which in turn means there can be far more entry/exit points. With buses and subways, the more stops you put in, the longer a trip takes.
The belts flow through tunnels that, as of 2280, are fairly drab and utilitarian compared to the rest of the colony. Eventually the tunnels will be clad in video displays that will make it look like you’re passing through living cities or landscapes on Earth. When you’re on a north/south beltway, unless you’re nearing one of the caps, the tunnel looks like it stretches ahead forever. By contrast, when you’re on an east/west beltway, the tunnel ahead curves up and out of sight.
The actual belts are alternating shades of gray so as to be easy to distinguish. Spaced out along the edges of each lane there are poles to grab onto to help keep your balance as you switch lanes. The outermost (fastest) belts are wider and have benches.
At the end of the north/south belts, the outermost (fastest) lanes are gradually eliminated so someone traveling to the end doesn’t bash into the wall. This isn’t necessary for the east/west belts, which run in a continuous loop around the circumference of the tube.
Hoppers
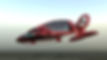
A hopper in flight.
Although the belts account for most travel inside the colony, air vehicles called hoppers are also common. Aurora only produces one civilian design at this time, a small four-seater. It’s about 2 meters tall and 3 meters long, with six ducted fans—two in front under the nose and four in back, two of which are gimbaled for directional control and forward thrust. Police hoppers are a modified version of the civilian vehicle. The military has a small fleet of custom hoppers that are available in the unlikely event of an invasion or some type of large-scale domestic disturbance.
Hoppers have directional active noise cancellation—outward facing when landing or taking off, downward when flying. This creates some odd effects: A hopper flying toward you will get quieter as it gets closer and go almost silent as it passes overhead. And if you're off to one side watching a hopper land, it will be loudest when it’s 10 meters off the ground, then the noise cancellation will redirect and it will get quiet as it completes its landing.
Hoppers fly autonomously. The flight path is seldom a straight line from one point on the tube to another. Due to the constantly changing Coriolis and gravity gradients inside the rotating tube, a straight-line flight would—counterintuitively—feel like a wild ride. Thus the hopper automatically adjusts its route according to passengers’ V-rating, a measure of how well an individual’s vestibular system resists queasiness. V-ratings tend to increase through age 5, then vary according to experience and physiological factors, but generally decline after age 35.
Planetary Ships (Civilian)
Broadly speaking, ships can be classified as either interstellar or interplanetary because their designs differ in fundamental ways. For trips within a star system like the Earth’s Solar System or the Barnard System, relativistic velocities (i.e., a significant fraction of light-speed) are impractical—they require too much time to accelerate and decelerate. Vessels designed for intrasystem travel (that is, travel within a system) are usually called planetary ships, even though almost all travel in the Barnard System is between colonies and asteroids or the moon Pogo, not between planets.
There are thousands of Auroran civilian ships. Most are involved in mining—either extracting and refining stath from Pogo and minerals from asteroids, or providing supplies and repair services to ships and equipment. There are also about 150 Navy ships, a few inter-colony shuttles and transports, and several hundred non-fusion powered ships for short trips to service the shipyard and Aurora’s hulls and to mine close-in asteroids. In all, there are about 1,500 landings and takeoffs every 24 hours. Ships land on the platform and are scanned for explosives, including nukes, then moved to Ascension Station where they are hangared and serviced. Ascension has two levels, one at 2,400 meters (above ground level), which is .4g, and another for large freighters at 3,200 meters, which is .2g.
When a ship needs only a minimal amount of service, it can also be unloaded and refueled in vacuum on the platform, without ever going into Ascension. However, inspection, repairs, and cargo handling are expensive in zero-g and vacuum. This may seem counter-intuitive, since weightlessness means that moving material, tools, and even oneself (be it man or machine) takes less effort. However, in reality, almost everything is more time-consuming, expensive, and dangerous. Every item, from individual nuts and bolts to tools to major pieces of equipment, must be secured at all times, otherwise the slightest knock will send it flying off into space (unless it hits another ship or the platform). Manned procedures must be performed in bulky suits. Every operator, be it man or machine, must somehow be secured to the working surface to get leverage. And any process involving fluids—refueling, hydraulics, even just lubrication—is prone to leakage from the extreme pressure difference with the vacuum. Therefore, about 80% of returning ships go to the hangars.
On any given flight, at the halfway point, the ship has to flip around and begin decelerating. Throughout this 20-second inversion, the fusion engine maintains a constant .25g acceleration while the plasma thrusters push the nose up and the tail down until the ship has rotated 180 degrees. The maneuver gives passengers the bizarre feeling of their body turning head over heels while gravity stays constant.
When the inversion is complete, the ship is still hurtling toward its destination but backwards. The main thruster, now firing toward the destination, has the effect of slowing the ship down. This also has the effect of providing the same one-quarter gravity, so the passengers still sit in their seats with their feet on the floor, just as before. But as a passenger, if you think about it, it’s confusing, because you know the ship has flipped around and the top of your head is pointed away from your destination, and yet everything feels exactly the same.
Planetary ships run a completely different fusion system than the interstellar transports. They use a different cycle (helium-3 and deuterium), are much smaller (about 2% the mass), and are far less efficient (0.1% of the exhaust velocity), but have a higher specific thrust, meaning they have a higher ratio of mass exiting the nozzles vs. engine mass. This translates into smaller ships that can accelerate faster but that can reach less than 1% of the velocity of the transports.
Ships are generally cylindrical for flight stability reasons. Their fusion engines are designed as modules that can be swapped out for servicing, so they come in just a few standard configurations. The largest ships, like freighters, may have as many as six engine modules. The engines sit at the base of the ships (as far removed from passengers and cargo as possible) and are roughly 5 meters in diameter and about 3 meters high, with notches for nozzle placement. Fuel tanks sit above them, in the shape of an inverted cone, providing shielding and absorbing waste heat which is used for fuel prep and electrical power generation. Stath shielding, shaped to match the inverted cone of the tanks, sits on the tanks. Everything else sits above the shielding. Unmanned fusion-powered ships can be as small as 7 meters in diameter and 9 meters tall, but more commonly they are about 10 meters in diameter and 13 to 20 meters tall. The big freighters that carry materials from the asteroids can be up to 20 meters in diameter and 60 meters tall.
An important factor affecting ship performance is mass (not including fuel). Reducing mass (of engines, payload, hull, and structural support) is the key to maximizing ship performance. One of the ways this is accomplished is by making a ship’s structure as strong as it needs to be and not more (including appropriate safety margins). In movies, we are used to seeing spaceships land horizontally, like airplanes. This requires that they have structural support across the length of their “belly.” But this only makes sense if they are making use of an atmosphere to slow down and land, as they might on a planet. If they’re landing on a stationary platform in space, it makes more sense to land vertically, with the engine slowing the descent.
When a ship is in flight, the only force acting on it comes from its engine. Even when it decelerates, it does so by flipping around, with the engine still applying force on the ship in the same direction as when accelerating (note: we are ignoring small lateral forces from retro rockets used for small maneuvers such as docking). Thus, ship designers do not need to support ships in a prone/horizontal position.
When ships are moved down to the hangars, they are subjected to gravity. But if they stay upright, the force from gravity is in the same direction as the force when their engines are on. Most planetary ships keep their maximum acceleration to .25g’s, but they are designed to handle up to .5g’s (military ships are designed for larger acceleration). That is why Ascension is 2,400 meters off the “ground,” where the gravity is .4g, and why ships land upright and stay upright through the entire transition process from the platform to the hangar and back. They are also never subjected to more than .1g’s in a horizontal or “up” direction.
Interstellar Transports
.jpg)
An interstellar transport with all its disposable fuel tanks still attached.
Interstellar transports are enormous. Each engine is 120 meters long, and there are six of them. There are 60 fuel tanks, each 88 meters long and 26 meters wide, in six rows of ten. The nose of the ship, including control systems and payload, is covered by a 120-meter-tall, 180-meter-wide foreshield designed to deflect and absorb interstellar dust and microparticles that would otherwise erode the ship’s hull. The fuel tanks are partially exposed but that doesn’t matter as they are discarded during the trip. In total, the ship is 540 meters long—about five football fields—and 180 meters in diameter, not including the main radiator complex at the tail.
Transports weigh 2,857,000 tons, 98.6% of which is fuel. The payload is “only” 4,000 tons, which not coincidentally equals Aurora’s annual quota for stath. Tanks, engines, shielding, and hull make up the remaining 36,000 tons. Compared to the mass of the fuel-laden ship, this may seem small, but 36,000 tons is roughly the displacement of five ocean-going naval destroyers or 420 Saturn V’s, the ship that took the first astronauts to the moon.
The transports are “slow.” In rocket parlance, the “fastest” ship isn’t the one that achieves the greatest velocity, but the one with the highest rate of acceleration. In this sense, interstellar transports are the slowest fusion-powered ships, accelerating at a stately .15g’s, about how hard you’d be pushed back in your seat if your car was going from 0 to 40 in 12 seconds. But they maintain this acceleration continuously for 18 months. The latest transport models in 2280 achieve a coasting velocity of 69,000 kilometers per second. In contrast, some of the fastest military ships sustain .6g’s, with bursts of up to 1.2g’s. The tradeoff is smaller engines and fuel tanks and short acceleration times. A typical planetary ship can achieve a maximum velocity of under 1,000 km/s.
Spaceships are governed by the rocket equation, which has just two variables—exhaust velocity (Ve) and mass ratio. Ve is a function of the sophistication of the fusion engine. Transports use an engine that employs a completely different fusion process than the one used on smaller planetary ships. The earliest version of the D3 engine—the general type used on the transports—was first tested on a ship in the 2060s and achieved a Ve of 700 km/s, a record at the time. In 2064 a ship achieved 6,000 km/sec using a variant of this engine, and it has been improved ever since and nearly perfected. The latest version has a Ve of 33,000 km/sec. Planetary engines, using a different process entirely, have a Ve of 340 km/sec.
Within the nose shield is a habitat for the 12 passengers. It consists of a 10 x 10, 120-meter-long enclosed truss connecting two habitat pods, each 15 x 20 meters to accommodate up to 12 passengers. The assembly rotates at 2.7 rpm, producing 1g in the pods. It provides 600 sq meters (6478 sq ft) living space for the passengers when they are out of hibernation. The truss has 10 levels as well, each with a different “gravity.” In total, they provide another 800 square meters of ancillary space for storage and some activities (some of the 10x10 meters at each level is consumed by support structure and gangways).
Interstellar transports dock at a shipyard that is detached from Aurora. It exists solely to service the transports, which are about 8 times the length of the largest freighters and, even if they could fit in Ascension, are too fragile to handle its 0.2g’s.
When transports were designed in the mid-2100s, fusion engine technology was still improving rapidly. The first transports made the trip from Earth to BS in 34.5 years. Within 20 years, that had dropped to 28 years, but progress has since slowed. In the subsequent 30 years, the trip dropped to 27 years, and in the last 30 years it has improved only to 26.5 years. It is widely assumed that improvements are approaching their theoretical limits. There are several reasons for this:
1) Relativity: The closer you get to light speed (c), the greater the effects of relativity. Mass gradually increases, so it takes proportionately more energy to accelerate. At 18% of c (the coasting velocity of the first transports), the mass effect is only 1.6%, meaning that a 10% increase in engine thrust would only deliver a 98.4% increase in velocity. At .23c (today’s transports), the effect has increased to 2.75%, so additional thrust is down to 97.25% effective.
​
2) Interstellar dust: Interstellar space is a near perfect vacuum, but there is still some cosmic dust, defined as particles ranging in size from individual atoms to micro-meteoroids. These particles, because of their high velocity relative to the transports, impart enough energy to be a problem, so transports require a dust shield. As their velocity increases, the mass of the shields also has to increase, further eating into any gains from better engines.
3) Technological maturity: As of 2080, fusion engines have become a very mature technology and improvements are more like tweaks with diminishing effects on velocity.
Moira and Pogo
​
Discovery and Naming
​
Moira is one of five planets orbiting Barnard’s Star, which is 5.9 light-years from Earth, making it the second closest star system after Alpha Centauri.

A photograph of Barnard’s Star taken by the Hubble Space Telescope in 2022.
Moira was discovered by Australian astronomer Jacob Penn in 2053. He named it after the lead character in On the Beach, an iconic post-apocalyptic novel written in 1957 by British novelist Nevil Shute.

Nevil Shute, author of 1957 sci-fi novel On the Beach.
The story details the experiences of a group of people in Melbourne as they await the arrival of deadly radiation spreading toward them from the Northern Hemisphere following a nuclear war. As the radiation approaches, each person deals with impending death differently. The novel was adapted into a 1959 film starring Gregory Peck, Ava Gardner, Fred Astaire, and Anthony Perkins.

Gregory Peck and Ava Gardner on the set of the 1959 film adaptation of On the Beach. Gardner played Moira.
Moira has one large moon, Pogo, named after the pogo stick that plays a major part in the character Moira’s story. In the mid-2100s, interstellar robotic probes dispatched by Pamex discovered large quantities of stath on Pogo.
Characteristics
Barnard’s Star is a red dwarf, a much smaller and dimmer star than Sol (the sun).

​Moira is approximately 3.2 times the diameter and 9.5 times the mass of Earth (9.5 Me). It is a small gas giant, about two-thirds the size of Uranus. Like Uranus and Neptune, Moira generates a little more energy than it absorbs from its star, so it “glows,” albeit weakly. It’s only faintly visible when viewed from an angle where it’s not illuminated by Barnard’s Star or by reflected light from Pogo. ​​

Photograph of Moira taken by a Pamex probe in 2129.
Moira is about 2.1 astronomical units (AU) distant from Barnard’s Star with a >9-year orbit. (An AU is the distance from Earth to the sun, approximately 93 million miles, or 150 million kilometers.) At this distance, its sun—Barnard’s Star—is very faint.
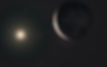
Photograph of Moira and Barnard’s Star, taken from Pogo.
While the basis for Aurora’s existence is the stath on Pogo, the resources for building and sustaining the colony come from the tens of thousands of asteroids orbiting Moira at the Lagrange points with Pogo. These asteroids’ proximity and shallow gravity wells make them easy to exploit, and much of the military tension and conflict among the colonies centers around access to them.
​
Stath
Discovery of Stath
Stath is shorthand for the element statheros (St), atomic number 126. Its unique properties make it capable of efficiently deflecting gamma radiation, which is necessary for shielding fusion engines.
Stath was first forged in particle accelerators in 2079, but could only be produced in microscopic quantities, so its use in industry was impractical and research on it was difficult. It was not known to occur in nature.
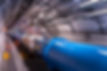
Large Hadron Collider particle accelerator.
Then, in the mid 2100s, interstellar probes discovered stath on the shore of a methane sea on Pogo in the form of crystals of StO4, called stathero-oxide.

A view from the surface of Pogo showing stath nodules on the shore of one of its liquid methane seas, with Moira in the background.
Stath Shielding
​
What makes stath so valuable is its ability to deflect high energy gamma and X-rays while absorbing almost no heat, making it ideal for shielding fusion engines. This ability stems from its extreme density and atomic properties. A single kilogram of stath can replace 200 kilograms of tungsten and reduce the “dry mass” (without fuel) of a typical ship by half or more. A warship with stath shields can accelerate twice as fast as one without; a freighter can carry two-thirds more cargo. Ships without stath became obsolete.
​
Stath Formation
​
No one is sure how stath forms naturally, how rare it is, and why it is sitting on Pogo in such large and exposed quantities. The leading theory is that it is created in the crust of neutron stars of a certain size, and is normally locked up forever by the star’s high density and resulting huge gravitational force. But if a neutron star has a collision or merges with another neutron star, parts of its crust could be ejected.

Computer simulation of two neutron stars orbiting each other in the process of merging and forming a black hole. The resultant explosion flings away some of the stars’ crusts.
Most of the ejecta would fall back onto the surface of the merged star, but some could be flung into space, where it could agglomerate into free-floating asteroids that could eventually collide with planets or moons like Pogo. If the asteroid was large enough, the impact could have distributed the material from the asteroid over a wide area.

An artist’s concept of a large asteroid striking a moon.
If the theory is correct, then stath is common on the surface of neutron stars, where it is inaccessible, and otherwise quite rare. Pogo could be the only depository within many light-years.
Scientists are still not sure why the stath is concentrated in Pogo’s methane seas in particular. One possibility is that a stath-laden asteroid hit Pogo during its formation, and so stath, as the heaviest element, collected at Pogo’s core, from which it eventually made its way to the seas via methane vents.
​
Stath Mining
Based on resource analyses from Pamex’s robotic probes, the Treaty of Bangalore divided Pogo into three zones, one for each colony, and established export limits of 4 tons annually.
Whereas the process of extracting and using materials from asteroids to build the tubes was already well understood, mining stath presented some novel challenges. One is that unprocessed stath coexists with several isotopes, some of which have short half-lives and are radioactive, which makes it destructive to materials and hazardous to humans. Therefore the unprocessed stath must be shipped off Pogo to specialized centrifuge waystations where the dangerous isotopes are separated and discarded. Only then can the stath be transported without additional shielding.
In addition, although Pamex’s probes initially detected stath on the shores of Pogo’s methane seas, follow-up missions determined that most of the stath was on the seabeds, at depths of at least 300 meters. These seas are similar to those on Titan, a moon of Saturn, but no attempt had ever been made to work below the surface of the Titan seas. Pamex scientists spent years developing mining equipment that could retrieve material from the Titan seabeds. (There was some hope that stath would be found there, but it was not.)
Conditions on Pogo’s seabeds are harsher than on Titan, so the robotic equipment would experience short half-lives. Even “normal” robotic miners required extensive maintenance, and autonomous repair systems could only do so much. It was thus decided that stath mining operations on Pogo would require a human presence, and the only practical way to do that six light-years away would be to establish a permanent colony.
​
There is relatively little heat from Barnard’s Star, so even during the “day,” surface temperatures on Pogo average −220° Celsius (53 K or −364° F). Pogo generates core heat from gravitational pressure, radioactive decay, and tidal forces, driving active thermal vents on the sea floors. (This is one theory as to how the heavy element stath rises out of Pogo’s core.) But the vents also spew an assortment of chemicals that worsen the already caustic environment of liquid methane that wreaks havoc on mining equipment.
Although stath mining efforts began almost immediately upon the colonists’ arrival, it was not until the fourth year that they were able to separate and process enough for the first transport back to Earth.
Population Challenges
​
The “Outpost” Challenge
​
Because a round trip takes more than 60 years, people who come to the Barnard System cannot realistically expect to ever return to Earth. Given this, the long-term feasibility of the Pamex’s stath-mining endeavor required, not just an outpost, but a complete society. The same was true for Hongzin and IMEC.
​
To develop a diversified and self-sustaining economy, a colony needs to grow to several hundred thousand people. But transporting that many people six light-years is prohibitively expensive and impractical.
Instead, each colony started with just a few hundred people, and all of them agreed to raise large families. In Aurora, after the first generation, the formal obligation no longer existed, but strong social pressures remain, and the government continues to offer significant financial rewards for raising children, including direct subsidies.
​
​
Growth Strategy
​
Each Original couple agreed to raise at least 12 children, and future generations were expected to raise at least six until the colony reached its minimum target population of 300,000.
​
What made this practical was artificial wombs. On Earth a small but significant percentage of women still opt for one or more natural births for various reasons—for instance, some believe it will strengthen their bond with the child, while others do it for religious reasons. But in Aurora, because the population is still so low (about 90,000 in 2280) and there’s so much work to be done, every adult is seen as a precious factor of production, and thus pregnancy is widely seen as an unnecessary health risk and a hit to colony productivity.
To ensure genetic diversity—which is vital for a healthy population—the original colony ship carried a million embryos in addition to the 400 colonists. As with pregnancy, raising a child who is genetically your own is legal but frowned upon, and people who do so are ineligible for most of the government benefits that come with raising children from the embryo pool.
Since Aurora began with just 200 couples, and there’s only a handful of immigrants each year from Earth and IMEC (as well as the occasional refugee from Hongzin), the colony needed to ensure that its population wasn’t dominated indefinitely by a few hundred giant clans. To this end, Aurora requires by law that children be given the last name of their embryonic parents from Earth. This means everyone in a family has different last names. (If someone has their own genetic children, a last name is selected at random, which is necessary to eliminate an incentive to having your own children.)
Population Profile


Highlights:
-
The growth rate was extraordinary compared to any society on Earth. For example, the population of the United States from 1950 to 1970—the Baby Boom—grew approximately 34%. Aurora’s population from 2060 to 2080 grew 330%.
-
The population quickly became dominated by young people. In 2280, just shy of 70% of Aurorans are under the age of 19, and 82% are under the age of 29. (For a discussion of electoral implications, see here.)
​
-
Starting in 2198, a transport from Earth has arrived every year, the primary purpose of which is to ship stath back to earth. But for the trip to Aurora, each ship also brings needed supplies and a dozen new colonists, selected for their specialized skills. For example, early transports carried a number of teachers—a vocation not included in the Originals.
-
In 2280—more than 80 years after Aurora’s founding—130 Originals are still alive.
-
Thanks to artificial wombs, there is no biological age limit to “having” kids. In addition, most people can expect to live well into their hundreds. It is not uncommon for Aurorans to “have” children in their sixties, seventies, and beyond. In fact, the 2280 census shows one second-gen under the age of 10 who is the child of an Original who is at least 110.
Governance
Structure
The Originals, as the first colonists came to be called, were all married couples. All had technical backgrounds. At least one spouse of each couple was military at the time of recruitment, and civilian spouses were inducted into the military at the commencement of training.
Initially the government consisted of a military council of 21 representatives, elected to staggered two-year terms by the 400 colonists before they left. Pamex selected a captain of the ship (who served as the head of the military after they arrived), a chief executive, and the heads of the government’s seven departments—Treasury and Commerce; Internal Security; Extraction; Manufacturing; Habitat (which included Transportation); Production; and Culture. Department heads reported to the Council and could be replaced at will.
Councilors serve staggered four-year terms, with half the Council up for election every other year. (The first group of councilors had terms of either four or six years so that they didn’t all face re-election at once.) Council positions are unpaid—they all have day jobs or are retired. The admiral of the military has a four-year term, is appointed by the chief executive, and does not report directly to the Council, although funding is controlled by the Council.
A document called the Charter serves as Aurora’s constitution and also defines its relationship with Pamex. The portions of the Charter that concern governance can be amended by two consecutive two-thirds votes held at least two years apart (i.e., separated by an election).
​
The “Kids” Problem
For the first 20 years, the number of adults only increased from 400 to roughly 640, from the 12 additional specialists sent with each annual transport. But once the “kids” (the second generation) started turning 21, they would quickly outnumber the adults, and the colony planners did not want to be in a position at year 24 where the 21-25-year-olds controlled the government.
So the Charter provided that starting in year 22 and for 24 more years, the Council would add one new member each election cycle, elected at large by all residents over 21, while the original 21 seats would be elected by voters 40 and older. Thus, starting in year 46, the Council had 33 members—12 “new seats” elected by all voters aged 21 and over, and 21 “old seats” elected only by those 40 and older.
Economy
​
Overview of Aurora's Economy
As outlined in the Charter, Aurora gets a guaranteed cut of the proceeds from the stath it ships back to Earth. (The size of this cut has become a major political issue, which is discussed here.) The colonists use this money to purchase whatever they need from Earth—and there’s a lot they need.
Purchases from Earth fall into two major categories: physical items that are shipped to Aurora on the annual transport (which is filled with stath for the trip back to Earth), and intellectual property, ranging from songs to medical technology patents, that are electronically transmitted from Earth.
The colony planners knew that it was crucial for Aurora to remain part of the world economy. For its 400 original colonists, Pamex needed to recruit the best and brightest, which wouldn’t be possible if prospective recruits expected the colony—which would be home to both them and their progeny—to be a backwater.
Aurora’s population, even after a century of rapid growth, would be miniscule compared to that of Earth, and thus would only be able to produce a miniscule fraction of humankind’s cumulative innovation. Consequently, access to intellectual property from Earth was—and remains—vital.
The colony ship, the Carifellan, carried a vast library of both classic and contemporary media, including literature, music, video, games, and other entertainment. It also had an extensive technical library including academic journals and rights to many patents. Although some of this IP was open-source, most had to be purchased, which meant the colony planners had to make tens of thousands of decisions about what to buy.
Aurora continues to buy IP from Earth on an ongoing basis, with each transaction taking 12 years—six for the order to reach Earth, six for the IP to be transmitted back. This time lag leads to some interesting dynamics (see "Economic Implications of Time Lag" below).
In general, given the enormous cost of interstellar travel, the only physical items that are worth buying from Earth are ones that are small and can’t be easily produced in the colony. Big-ticket purchases include:
-
Probon starters, which are consumed every time a ship’s fusion engine is ignited, and so are necessary for all spacefaring activity. Probons—antimatter protons—can only be produced in huge particle accelerators on Earth, and Aurora pays billions of dollars a year for them.
-
Millions of specialized chips necessary for the colony’s sophisticated equipment.
-
Prescription drugs.
Finally, Aurora also spends a large amount of money on maintenance and improvements of Homer (see below), the enormously complex IA (intelligent agent) program that Aurora relies on to represent its interests on Earth.
​
Transitioning to a Market Economy
In its early years, Aurora was basically a military base, but the plan was to quickly transition to a more traditional civilian model. The captain of the colony ship became the head of the Ministry of Defense, which was a branch—albeit semi-independent—of a government run by an elected council. A core responsibility of the Council was to manage the incremental privatization of the economy.
At first, everything from food to housing was provided by the government, and accordingly every colonist was temporarily taxed at 90%. They could either save their take-home pay, spend it on nonessentials like booze, or even buy things from other people.
The assumption, however, was that government-run distribution of resources was inefficient and unpleasant because everyone had different interests and needs and they didn’t all want the same things. So as production increased and diversified, the Council gradually lowered the tax rate and converted products and services from “free” (tax-funded) to buyable. For example, after initially only having a mess hall, the colony opened its first grocery store, and from then on you could either buy groceries or pay to eat at the mess. Both the store and the mess remained government-run, with prices set by a committee that factored in cost to produce, availability, and demand.
The next phase of the economic plan called for a transition from government to private production. The Charter provided a comprehensive set of rules and procedures to facilitate privatization. Anyone could start a part-time business out of their home. Some of the first things privately sold were homemade candles, snack foods, and art. But if you wanted to start a “real” business, you needed to submit to the Council a JMP—a justification and mitigation plan.
Few JMPs were submitted and none approved for 20 years because the population was so small there wasn’t much of a market and every single person’s job was essential. The first approved plan was submitted by Ariel Pereira, a mechanical engineer who’d figured out a way to improve the colony’s ultrasonic welding machine, which made absorbent products ranging from diapers to industrial spill pads.
The colony had two such machines and he proposed to purchase one of them. The colony would continue running the other, thereby addressing the Charter’s rule against granting a monopoly on existing essential products. Pereira would be required to meet certain production quotas to avoid shortages. He also agreed to be responsible for training recent college grads to take over his duties as one of the colony’s industrial robot maintainers. The Charter protected Pereira by prohibiting the government from reducing its prices unless there was a decrease in its direct production costs.
A decade later Cindy Ashcroft filed a JMP asking the Council to give her the mining rights to two asteroids along with the robotic mining equipment already in place there. She guaranteed the same production levels while paying off the equipment over many years. Her company, Valence, was quickly successful and eventually expanded to scores of asteroids. One of her secrets, it later emerged, was what she didn’t do. In the annual transports from Pamex, the Navy received upgrades to its mining equipment and dutifully installed them, which required the equipment to be rotated out of service for several weeks. Ashcroft, a Navy mining engineer, believed the incremental benefits from most of these “upgrades” did not justify the downtime, and worse, they often created problems of their own. She significantly increased productivity by forgoing some upgrades entirely and holding off on others until several could be installed simultaneously.
​
​
Economic Implications of the Time Lag
The enormous distance between the colonies and Earth—approximately six light-years—makes for some unprecedented economic dynamics. A round trip (which no one has ever done) would take more than 60 years, and electronic correspondence takes 12—six for a message to get there, six for the reply to come back.
Imagine you own an Auroran pharmaceutical company and you want to import drugs from Earth. When you look at the catalog, it’s six years out of date, and by the time your order reaches Earth, another six years will have passed. In those 12 years, prices will have changed and better drugs will have been developed. How, then, can you make sure you get what you want and at the best price?
Theoretically you could hire a representative on Earth, but you’d have no personal relationship with them, and it would be hard to stop them from striking under-the-table deals with drug manufacturers to overcharge you.
The solution is Homer (see below).
​
​
Homer
Homer is an enormously sophisticated intelligent agent (IA) system responsible for conducting Aurora’s business on Earth. Both the Auroran government and private businesses can feed Homer parameters for what they want to purchase, and then Homer either conducts auctions or negotiates for the best possible deal.
For example, say the Auroran government wants to buy movies from Earth studios that it will then sell on the colony. It tells Homer it will pay $0.40 per view for movies that exceeded a certain gross receipts threshold on Earth and appealed to a demographic close to that of Aurora. Homer advertises this request and then engages with studios to get the best deals it can.
The entire exchange of commerce between Aurora and Pamex depends on the colony’s confidence that it has complete control over Homer. Per agreement, Homer “lives” in computers in a secure facility in the Pentagon, and communications between Aurora and Homer are quantum-encrypted. Homer monitors its own security and provides continuous updates on its security status to the colony.
It was originally set up and programmed by a team of six of the Originals, and is now maintained by a department of 17 software engineers on the colony. In addition, there is a staff that provides support for “users,” such as the owner of a pharmaceutical company who wants to give Homer parameters for buying certain drugs.
In addition to managing purchases and auctions and monitoring its security status, Homer:
-
seeks to prevent price fixing in auctions by performing intelligence operations and collaborating with law enforcement and antitrust officials
-
runs the secure high-bandwidth communications system to Aurora
-
acquires all the free IP it can and prioritizes the content for transmission to Aurora
-
performs intelligence gathering on the military plans and capabilities of both Pamex and other Earth powers
-
performs commercial intelligence gathering on trends in a wide variety of industries
-
can use Auroran financial resources to hire specialists, such as lawyers and engineers, to do any of the above as well as to perform physical maintenance if necessary.
Politics
Origin of the Griffins and Turners
When the colony ship arrived in the Barnard System, there were no political parties. The colonists had selected the members of the Council before they left, and the first elections were held in the fourth and sixth years after arrival.
Council meetings had a kind of internal contrast unlike anything found in democracies on Earth. On the one hand, they were “small”: with only 400 colonists and 21 councilors, everyone knew each other, so there was an intimate, usually congenial atmosphere. On the other hand, these meetings were “big”: the colonists were in the process of building a whole new society, and the decisions they had to make were on par with those of national legislatures or heads of state.
In the first few years after Aurora’s founding, a long-running debate came to a head. Thanks to Pamex’s efforts to keep its stath discovery secret for as long as possible, it had a nine-year head start on China’s colony fleet. Consequently, it had had the luxury of not having to send warships along with its colony ships, since when the colonists arrived, they would have nine years to build up their navy before any threat from another colony arrived.
​
China, by contrast, did have to send warships—at great cost—because Aurora’s warships would be waiting for them.
The issue in front of the Council was how powerful the Auroran military needed to be. They already had a small navy: 12 Cutlass-class ships that had been built with the dual engines of the six Avant ships from Earth, and one larger frigate using the backup reactor from the colony ship, the Carifellan.
Pamex had sent Aurora all the intel it could gather on the capabilities of the coming Hongzinese navy, and the existing Auroran navy had been built accordingly. Some Aurorans, however, emphasized that there was no guarantee the intel was completely accurate, and given that Aurora was all alone in deep space, they couldn’t afford to underestimate a potential enemy. These Aurorans wanted to divert more resources into strengthening the navy.
Other Aurorans felt that doing so would risk inflaming tensions and touching off an expensive arms race. And of course Aurora had limited resources, so any efforts to strengthen the navy would detract from the myriad other projects involved in starting a whole society from scratch.
Leading the more hawkish faction was Councilor Jakeem Griffin, a prominent Navy captain. The doves were led by Julianne Turner, a medical robotics technician who happened to live next to Griffin (with only 200 couples on the colony, this wasn’t that extraordinary).
Although the debate was intense, the population was still tiny, everyone knew they had to get along regardless, and so both sides were friendly—an atmosphere that first- and second-gen colonists still reminisce about. The two sides began calling each other the Griffins and the Turners, and the terms stuck, both sides a little proud of the fact that the names were lighthearted. Today’s parties are named after those two neighbors from 77 years ago.
Griffins and Turners
​
The two main political parties in Aurora are the Griffins and the Turners.
One of the main fault lines between them is whether the colony should be devoting relatively more to military or domestic spending. In one way, this is similar to the split between major parties in many developed countries on Earth. However, a major difference is the colony’s limited manpower. When there are so many essential jobs to be done and so few adults on the colony, every tradeoff is felt much more acutely. If you decide to build a naval vessel based on a new design, that requires hundreds of engineers and other specialists who can then no longer spend their time on mining ships.
The Griffins emphasize the importance of economic growth, colonial self-reliance, and a strong defense. Growth will lift living standards for everyone. Self-reliance is essential to break Pamex’s stranglehold on Aurora’s economy. They stress the colony’s military vulnerability to Hongzin, China, and even potentially Pamex.
The Turners generally agree the colony still needs to grow to achieve higher living standards and that hard work and maximizing productivity are key to accomplishing this. But they also believe the nature of the colony is gradually changing, transitioning from an intense, growth-driven frontier culture to a more “modern” Earth-like society. They generally favor more emphasis on education, social services, and reducing inequality. They believe this requires a good relationship with Pamex.
The other big fault line between the Griffins and Turners is the price of stath, which is discussed here.
Walkers
The Walker party is named after Angel Walker, one of the original 400 colonists, an agronomist and later economist and philosopher who advocated for making a transition to a less “achievest” society, with more stress on culture and reducing economic disparity, less on competition and growth. She was on the Council for eight years, retired 19 years ago at the age of 107, and passed away two years later. She was warm and compassionate and a gentle advocate, well liked by friends and foe.
Her ideas never reached the mainstream while she was alive, but she has been rediscovered by young people, mostly fourth gens and some thirds. Raised by competitive parents and on a steady diet of Earth-based drama and news shows emphasizing all the problems “back home,” they argue that the emphasis on competition and growth are what’s wrong with Earth, and that Aurora doesn’t have to make those same mistakes. Specifically they argue for suspending new privatization, reversing the privatization of power and communications utilities, eliminating private schools and health services, and banning competitive sports in schools.
While most Walkers are interested in gradual change, a few radicals have staged demonstrations at corporate headquarters protesting what they see as overly cozy or corrupt Council-granted business licenses. Others have demonstrated during semi-pro games of bolo, a zero-g sport that is attracting more and more fans and media attention.
The Stath Debate
​
When Aurora was being planned, it wasn’t known exactly what the price of stath would be, since it had never been produced in large enough quantities for any practical use. It was projected that the market price would be $18,000 per kilogram. Aurora was guaranteed $4,500/kg (25%), which would be enough for the colony to build out its infrastructure and prosper. The remaining $13,500 would go toward transportation and Pamex’s profit. The Charter fixed these prices for 100 years from receipt of the first shipment.
For the first several decades after Aurora’s founding, Pamex sold its stath to shipbuilders via contracts that prohibited the companies from reselling it or in any way disclosing its price. When IMEC (the Indian Mining Enterprising Colony) was established, India created an open market, and the price turned out to be around $28,000/kg.
This sparked outrage in Aurora—it meant Pamex had secretly been making a profit much larger than the Charter’s projections. The debate over how Aurora should respond is ongoing.
Hardline Griffins want to threaten to start selling stath through India’s open market unless Pamex grants the colony a bigger cut of the profits.
Turners argue that this could provoke a military response, but Griffins counter that this is unlikely for several reasons:
​
-
Building an armada to seize control of Aurora would be exorbitantly expensive.
-
Any armada capable of overwhelming Aurora could overwhelm Hongzin too. China would never allow this without a big fight.
-
The armada could not be robotic, since keeping the mining operations running requires people. Once the armada arrived, those people’s interests would align more with Aurora than with Pamex. Thus, the armada might well end up allied with the colony.
Turners generally concede that a military takeover is unlikely, but that doesn’t mean it’s impossible. As Councilor Georgette Ridere once put it: “Griffins say the odds of a Pamex attack are low. I agree. But when someone asks if I want to gamble with my family’s lives, I don’t say, ‘What are the odds?’, I say, ‘Fuck off.’”
Furthermore, the Griffins’ plan is entirely dependent on India. If India refuses Auroran stath for whatever reason (for example, a new regime decides to prioritize relations with Pamex), Aurora would lose all its leverage. In fact, given the six-year communication lag, this could have happened already.
Finally, Turners point out that Pamex never violated the Charter. Although the Charter’s locked-in price for stath ended up favoring Pamex, it could have turned out the other way around: if the market price of stath had fallen short of the projections, that price guarantee would have been key to the colony’s economic survival. So the argument that Aurora is being short-changed ignores the risk Pamex took in agreeing to a price guarantee, and ignores the value of the security the guarantee provided.
Until recently, the Turners’ position enjoyed grudging public support. But as the colony’s economy has grown less dependent on Pamex, and as more and more young people with no real attachment to Earth reach voting age, public opinion is shifting toward the Griffins.
Hongzin
History of China and Hongzin
China—which, after wars in the late 21st century, came to include Nepal and Mongolia—has a loose association with much of Africa and the Middle East. It has military parity with Pamex. The Great Reform of 2137, led by the now legendary General Chao Hua, occurred after 25 years of economic stagnation. In a bloody revolt, Chao and the military ousted the corrupt and widely derided Central Committee and installed a military tribunal under her control. She proceeded to loosen control over the economy, reigniting growth, and loosened some controls on political freedom, allowing freer exchange of ideas both domestically and with the rest of the world. Chao was also aggressive militarily, and the Shipyard War was fought under her rule. She spearheaded the design and construction of the Hongzin colony flotilla.
Hongzin is anglicized shorthand for the full name, HóngxÄ«ng ShÄ›ng, which means Red Star Province. Unlike IMEC, which is commercial, and Aurora, which started out run by the military but transitioned to democratic civilian control, Hongzin is still controlled by a military triumvirate—a president who heads the military, which is called the Chinese Colonial Navy (CCN), and two vice presidents, who head the Central Production Command and the Cultural Commission. Technically they are elected by a Central Council, which has 40 representatives, elected from the three divisions of the government—20 from the Navy and ten each from Production and Culture. While the CC elections are ostensibly democratic, all nominees require approval by the Triumvirate, a circular process that ensures control by the Triumvirate. This is all dictated by The People’s Charter.
By virtue of Pamex’s success in keeping its stath discovery secret, China’s colonial efforts lagged behind those of Pamex, and their fleet didn’t arrive in the Barnard System until nine years after Aurora. Based on the terms of the Treaty of Bangalore following the Shipyard War, Hongzin had 15 years to stake claims to asteroids, which entailed establishing and maintaining mining operations. Its strategic plan was to use many small robotic mining systems to maximize the number of asteroids under its control, at the expense of efficiency and production due to a lack of scale at any one site. It also placed little emphasis on nonessential production, embracing a spartan culture in which people took pride (or at least, were supposed to take pride) in needing nothing beyond necessities.
Hongzin’s population growth model was influenced by China’s experience in the late 21st century. Facing an aging and stagnant population, China experimented with birthing large numbers of children—estimated to be more than 200,000—from artificial wombs and raising them entirely in state-run programs, without parents. Despite attempts to keep standards of care high, the result was high percentages of disaffected and poorly educated young adults who were antagonistic to the state. Thus, it came to be accepted that raising youngsters without parents is not a good idea, so Hongzin incentivizes large families just like Aurora does.
Hongzin's Relations with Aurora
By 2233 (Hongzin’s 35th year), the number of young adults was double that of the original colonists, and there was growing dissatisfaction with their lifestyle and freedom compared to what they knew about Aurora and IMEC. Hongzin leadership attempted to employ a tough-love strategy of heavy-handed control and propaganda while grudgingly increasing consumer production.
During this period the colony became more aggressive militarily, mainly with respect to Auroran-claimed asteroids. Disputes with Aurora arose, stemming from varying interpretations of the Treaty of Bangalore’s treatment of large asteroids: Hongzin challenged Aurora’s position that whoever starts mining a portion of a large asteroid has rights to the whole body, even if it is only mining a small portion of it. Between 2235 and the time of the Koitan Skirmish (see below) in 2272, there were more than a dozen incidents involving live fire and casualties.
​
From the beginning, Hongzin has been Aurora’s largest concern. It is a closed and hostile neighbor, with grievances—some arguably justified—toward both Pamex and Aurora. On Aurora, the semi-official line is that Hongzin manufactures provocations to stoke nationalist sentiment and distract from domestic problems. Hongzin claims much the opposite—that the Auroran Navy regularly provokes Hongzin to protect its own power and budget in the civilian government.
Both sides, as well as IMEC, deploy a MAD (“mutually assured destruction”) strategy, maintaining an arsenal of hopefully never-to-be-used weapons along with aggressive surveillance. They also maintain, even in times of active conflict, channels of communication designed to prevent misunderstandings. This has been the nature of conflict since the advent of nuclear weapons, but the necessity for these precautions is amplified out here, where all the colonies are fundamentally fragile, and the weapons, which include Javelins, sophisticated biological and chemical weapons, and fully autonomous delivery systems, are more fearsome than ever.
The Koitan Skirmish
​
One of the non-Trojan asteroids where Aurora set up a small robotic miner was named D1712, the D standing for the fourth orbital band outside Moira’s orbit. The asteroid is an irregular shape, like a tangerine sitting on a mango. It is roughly 4 kilometers long from end to end and has large concentrations of heavy metals compared to other non-Trojans.
Hongzin decided to stake a claim as well. It parked a largeish mining ship on a flat part of the surface, 2 kilometers from the Auroran miner, and named the asteroid Koitan.
Aurora informed Hongzin it considered the operation illegal and sent two corvettes to blockade it. Hongzin denounced this as piracy and sent four ships to another Auroran-claimed non-Trojan that it named Heitan, forcibly removing the robotic miner there and installing its own facility.
Aurora responded by forcing the surrender of the two Hongzin staff on the Koitan facility and destroying it.
Up to that point, they had refrained from sending fighting ships to the same place at the same time—a familiar dance. Neither could afford an all-out war, and both knew it. But then a robotic Hongzin corvette launched two rockets and destroyed a manned Auroran corvette on its way to Koitan. Two Auroran corvettes pursued the Hongzin drone and destroyed it. Aurora sent larger sorties to both Koitan and Heitan.
IMEC mediated and convinced the two sides to agree to a three-day truce and to meet. During negotiations, it was announced that Hongzin President Hoa had been ousted, and the new Central Council president, Admiral Zhao Li Jing, said she was withdrawing from the talks but would agree to extend the truce for an additional seven days. No other information was provided. The situation was never resolved: to this day, Aurora maintains control of Koitan and Hongzin’s mining equipment there and holds the two Hongzin miners captive, while Hongzin holds Heitian. Both sides can plausibly claim they achieved some success. The Koitan Skirmish, as it came to be known, was the closest the two colonies have come to outright war.
IMEC (the Indian Mining Enterprise Consortium)
History of India and IMEC
​
Aggressive nationalism and militarism accompanied India’s rapid economic growth in the late 21st century, culminating in the 20-Year War, a complex hot conflict involving both Pakistan and China over territorial rights to Kashmir and Tibet and maritime rights in the Indian Ocean. In 2105, China and India settled, leaving a diminished and exhausted Pakistan on the sideline. China assumed complete control of Tibet while India took all of the Kashmir region.
By the 2120s, India had a newly influential military, but was striving to be neutral in the usually contentious relations between Pamex and China. A series of centrist governments encouraged private orbital development, and several very large space-based mining and solar power companies emerged. With less government involvement in space endeavors, India tended to lag behind China and Pamex in development of mega projects like interstellar-class fusion engines and the huge O’Neill cylinders. Its approach was to piggyback off the large-scale R&D of others.
​
India helped broker the Treaty of Bangalore, which ended the Shipyard War, and secured for itself a share of stath mining rights on Pogo. Rather than forming its colonial efforts around the military, as did China and Pamex, India established a private consortium of its largest mining companies, which came to be known as the Seven Sisters, and they formed the Indian Mining and Extraction Consortium, or IMEC.
​
IMEC didn’t reach the Barnard system until 2215, three years after Hongzin and 12 years after Aurora. Its design was a two-thirds scale copy of Aurora. Like the other colonies, IMEC started with just a few hundred colonists, but organizationally it was decentralized, with control over core operations such as asteroid mining, stath mining, and construction apportioned to divisions of the Seven Sisters. As the colony grew, it encouraged smaller, independent vendors to provide other products and services. Over time, these “peripheral” goods and services grew to be well over two-thirds of the colony’s economy.
Similarly, IMEC’s government was run by IMEC corporate, controlled by the Seven Sisters, but it created elected advisory bodies. Over time, these gained clout and effectively ran most government services, despite being nominally under the control of corporate IMEC. This system of somewhat tangled structure and mixed accountability is prone to corruption and has led to a higher degree of income inequality than in Aurora or Hongzin.
​
But IMEC also has the highest average standard of living of the three colonies. This is partly due to its more decentralized and privatized economy, but also because the money it receives for stath is substantially higher than what the other colonies earn—almost $9 billion per year more than what Aurora gets, which would amount to about $100,000 per capita on Aurora. That does not all translate directly into standard of living because all the colonies are making substantial investments in tube construction to accommodate population increases, but the difference is nonetheless substantial (it is also difficult to make comparisons between IMEC and Aurora because the IMEC was established later and has a smaller population and is building a smaller structure, at least initially).
​
IMEC’s standard of living also benefits from a smaller share of resources devoted to defense.
​
Relations with Aurora and Hongzin
While IMEC maintains strict neutrality in the tension between its aggressive neighbors, it must also ensure that neither sees it as an easy target. It is, of course, under India’s umbrella, but given how far away Earth is, this mainly serves to protect it from actions by one of the other Earth alliances. Locally, IMEC knows its neutrality and smaller defense footprint potentially make it look weak. It must maintain at least enough defense to make the cost of aggression higher than the benefit. To show that it is not completely averse to conflict, IMEC is aggressive about mining claims and has a history of responding decisively to any perceived encroachment on asteroids or its stath zones on Pogo.
The Threat from Earth
​
An attack from one of the Earth alliances, or even two or more of them acting together, is always a possibility. Earth has immense resources and, regardless of the time and distance, could create vast, possibly robotic armadas against which there would be no plausible means of defense.
But an attack from Earth must overcome several obstacles. First, any ship that can make the six-light-year trip in less than a generation will be weak and vulnerable as a warship. Using any plausible fusion technology, such a ship would have a huge mass ratio. The transports used to ship stath back to Earth are already 98.6% fuel. There is no practical way to significantly increase the mass ratio—engines, fuel tanks, shielding, and payload cannot be cut to zero. This necessarily means:
​
​1) low-mass engines with high Ve (exit velocity) will generate low thrust, and even if they had more thrust, large mass ratios dictate fuselages that cannot withstand high-speed maneuvering. Hence these ships would have little maneuverability;
​
2) lightweight frames and minimal shielding will make them delicate targets; and
​
3) they would spend the last months of the trip slowing down, during which time their nozzles would be pointed at the destination, serving as brilliantly lit beacons announcing their arrival months in advance.
​
It would be a little like giant oil tankers retrofitted with weapons going into battle against destroyers and corvettes.
Because such transports themselves are sitting ducks, the only practical way to use them to launch an attack would be to:
1) send enough of them to carry a large fleet of much smaller, more agile attack ships—enough to overwhelm whatever the target colony has built for its own defense;
2) approach at an angle large enough that the exhaust plume would not be detected by the target; and
​
3) unload the smaller ships in secret some distance away.
This strategy has its own problems. First, the assembly of a fleet of these gigantic transports could never be done in secret, so the target colony would have a minimum of about 24 years to prepare. The Earth alliances could repurpose their fleet of stath transports, which are similar in size, but this would disrupt the supply of stath for many years, presumably the opposite of their goal. Second, any gap in the frequency of transports would be obvious to the colonies. The colonies would thus have at least 24 years to prepare, probably closer to 30 due to the time needed to assemble and modify the ships
There is another challenge that is even more fundamental. Assuming the goal of an attack is not to destroy the colony but to gain control of stath mining, an attack would need a human contingent to negotiate surrender and administer the newly subjugated colony. It could not be completely robotic. But any crew would know that, due to the length of the trip, they would be staying in the colonies permanently. Their interests, therefore, would really align more closely with the colonies than with Earth. While an attacking alliance could take steps to ameliorate this problem—e.g., perhaps by holding families on Earth hostage—it could not have a high degree of confidence in the loyalty of the invading force.
This loyalty issue is made worse by the fact the invaders would know that combining forces with the colony would presumably more than double the size of the colonial navy, making any attempt by Earth to launch another attack in the future many times more costly and difficult. Another issue is that if the two sides fought and both suffered substantial losses, the victors might be so weakened as to be unable to defend itself against the other colonies.
Finally, compounding all these issues is the fact that an attack by one alliance would necessitate a response by the other alliances. They could not afford to allow for the possibility that an invading fleet could either attack a different colony or succeed in its invasion, possibly resulting in the subjugated colony having a substantially larger fleet than the other colonies.
So the three major Earth powers could agree to coordinate or combine their attacks, but barring a thoroughly combined command structure, the risks of mischief between the powers in the 30+ years of travel would be very high.
The real risk to the colonies is their unusual economic dependence on Earth. They need its intellectual property, both for short-term requirements, such as new medications and electronics, and for the progress that accompanies the flow of innovation from tens of millions of engineers and scientists six light-years away.
Military Tech
​
Lasers
​
Lasers are in some ways the perfect space weapon. A laser beam moves at the speed of light and, with no atmosphere or weather to contend with in space, can be devastating at fifty or even a hundred thousand kilometers.
But every weapon has its limitations. A warship’s 250-megawatt laser can burn a hole through 19 centimeters of titanium shielding from 20,000 kilometers away in two seconds. But due to energy conversion inefficiencies, that would require the full output of a ship’s fusion engine, keeping the ship from maneuvering while firing and consuming valuable fuel.
Also, the fact that burning the hole would take several seconds means that the laser would have to stay focused on the same exact spot of the enemy’s hull. This is difficult to do at 20,000 km, due to jitter (random vibrations on both attacking and defending ships), the spread of the radar signal over that distance, and the 0.133-second duration of the signal’s round trip.
There are several ways to defeat laser attacks. First, specialized graphite shielding is, pound for pound, almost twice as effective as metals. But graphite is brittle and of less use against missile strikes.
The most effective countermeasure is to rapidly rotate the hull so that the laser cannot track the same spot on the hull, no matter how good its targeting information. This requires both a nearly instantaneous response and extreme rotational acceleration. The instant a laser strike is detected, the ship automatically fires a set of “charge thrusters”—typically four directed explosive devices, arranged around the circumference of the hull, that provide the thrust to produce almost instantaneous rotation.
Humans cannot survive such g-forces, and even if they hypothetically could, they would be incapable of working in a rapidly spinning environment. If you did want to have a manned ship that could use anti-laser rotation, it would have to have an external shield that would spin around a non-spinning hull, which would be complex, fragile, and very heavy. This is one of several reasons that fighting vessels are almost all unmanned.
Unmanned military ships are able to engage in defensive and rapid attack maneuvers autonomously, but when they are more than 100,000 km from Aurora or an Auroran base, where the two-way comm latency increases to two thirds of a second or more, they are accompanied by a heavily shielded command vessel that can digest battle space information and provide tactical and strategic direction.
Manned command vessels still need a way to defend against lasers, since rotation won’t work. They use a liquid radiator system, in which small “capillaries” circulate liquid coolant through the shielding. The liquid absorbs the heat from the laser strike, vaporizes, and is vented into space. This significantly increases the time needed for the laser to penetrate, giving the ship more time to maneuver. The whole system is heavy and complex, making these manned ships even bigger and slower. Because there is no real “behind the frontline” in space, duty on command ships is amongst the riskiest in the Navy.
Missiles
Unlike “dumb” projectiles, missiles are self-propelled and guided, meaning they can change direction in-flight, either by ship command or in response to signals from their own sensors. With their maneuvering capability and small size, they can often evade laser defenses. Because they have a large thrust to mass ratio, they can accelerate quickly, whereas a bulky ship with its large fusion engine takes a while to change course—think of a torpedo vs. an aircraft carrier. A missile requires a relatively long time to reach the target—fired from a distance of 20,000 kms, it might take more than two minutes, and initially there would be a delay in the targeting data of 0.13 seconds—but as it gets closer, the delay melts away to nothing. This can allow the missile to target specific ship segments, such as lasers and engines. Comparable precision is difficult for lasers because at a distance, their targeting is not accurate enough, and at close range, the large relative motion of the two vehicles combined with spinning countermeasures allows for little time on target.
Missiles have downsides. Their engines make them easier to detect. They are expensive compared to the per-shot cost of lasers, and they are substantially larger, so a ship can only carry a limited number. They also carry limited fuel. And while they can outmaneuver a target at close range, they can only accelerate for so long. A fool-proof defense against missile attack is to turn on the fusion engines long before the missile arrives and slowly pick up relative velocity until the missile can’t catch up. Of course, that involves running from the attacker, and consumes valuable fuel. In fact, using a missile to force an opponent to “run” is one of its principal uses.
​
The Impracticality of Projectile Weapons
In space combat, opposing ships rarely come within even a thousand kilometers of one another due to their vulnerability to lasers and guided missiles. At these long ranges, unguided projectile weapons are of limited value. Take, for example, a railgun that can fire projectiles 10 kilometers per second (for comparison, a typical high-velocity fighter jet or tank cannon has a top muzzle velocity of less than 3 km/s.) If a target ship is 1,000 kilometers away, and assuming it detected the shot, it would have 100 seconds to simply get out of the way of the unguided projectile. Combined with a railgun’s large mass, it is an ineffective weapon for a ship.
However, the colonies have large railguns mounted on stationary platforms near the tubes. They are primarily used against asteroids, but also serve as the last line of defense against missile attacks.
​
​
Particle Beam Weapons
​
Particle beam weapons fire streams of atomic particles at very high (but sub-light) speed. Particles can be electrons, neutrons, alpha particles (helium nuclei), or heavier ions. These weapons are similar to lasers in that they are effective at long range. They consume much more energy than lasers but degrade surfaces more rapidly. The problem is that, as of 2280, they are physically too large for the ships the colonies are building. Development of smaller, lighter systems is an active area of research on Earth.
​
​
Macron Accelerator (a.k.a. Dust Gun)
​
This is a version of a particle beam weapon, but instead of firing ions or atomic particles, the projectiles are “macrons,” manmade bacteria-sized particles with diameters on the scale of a micron (micrometer), which is 1/1000th of a millimeter, or 1/40th the diameter of a human hair. A charge is applied to the macrons' surface and they are accelerated using the same principles as an ion engine, railgun, or particle accelerator.
​
Due to their size, they have a large surface to volume ratio, which allows for a large charge per mass. This means they can achieve velocities of up to 1,000 kilometers per second—100 times as fast as a missile—with a “gun” less than 10 meters long. This would make them intriguing weapons, except that even with firing rates as high as a million particles per second, they do not deliver enough energy to be useful. What intrigues scientists is the idea of using hollow macron tubes filled with isotopes such as deuterium that would undergo fusion when the projectile implodes on the target, releasing large amounts of energy. Development is ongoing.
​
Nukes
Nuclear weapons don’t work the same way in a vacuum as they do on Earth. If they detonate in space, their blast and thermal effects disappear since there is no atmosphere to propagate either.
But they do still emit radiation, and in fact nuclear weapon intended for use in space are designed to produce more radiation and less blast. This is what a so-called “neutron bomb” is. With no atmosphere in the way, radiation propagates perfectly through a vacuum. But its effects still fall off rapidly as the square of the distance (radiation at distance d = radiation at the source divided by d^2). A nuke can be very effective against a manned ship, depending on yield and how close it gets. Typically it needs to be within about 100 km to create significant radiation exposure problems. But robotic ships with radiation-hardened electronics would suffer few ill effects. This is yet another reason that manned warships are vulnerable.
If a nuclear warhead detonates while in direct contact with a ship’s hull, the effect of the blast and heat can be catastrophic. Even though only a fraction of the concussive and thermal effects is likely to be on the target (most would be dissipated off into the vacuum), it would still likely be enough to punch a large hole in the hull and melt a lot of stuff inside. Keeping warheads away from the hull is a priority of defensive weapons and maneuvering.
One of the biggest risks faced by all the colonies is that of a nuclear device being snuck into a tube and detonated there. The effects of a blast from a small 10-kiloton device would be severe. If detonated in a lower service level, it would puncture a 200-300-meter-wide hole in the hull. If detonated on ground level, it might only cause a small hull rupture but would result in the immediate death of 3-6% of the population, serious injury to another 5-10%, and huge radiation fallout that would be difficult to handle. A 100-kiloton device (still not that big) would probably result in immediate decompression, and even if it didn’t, thermal and radiation effects would likely result in almost 100% casualties in the tube. People in the caps would have some chance of survival.
The colonies thoroughly scan all arriving ships on the landing platform for the signatures of nuclear weapons—radiation or radiation shielding.
​
Javelins
One of the unintended consequences of fusion engines was the potential to create weapons far more frightening than nuclear weapons.
If you took a big interstellar transport (say, 40,000 metric tons dry mass), filled it with fuel, and used all of it to accelerate (rather than saving some for deceleration), it could achieve velocities of about 1/3rd light speed—100,000 kilometers per second. If it slammed into Earth at that speed, it would have about 400,000 times the energy of the largest hydrogen bomb ever made. Scientists theorize that a single such strike would end all life on the planet.
On top of its destructive power, the speed of such a missile would make it difficult to detect and intercept.
​
Fusion-propelled missiles of any size are called Javelins. They are banned under international law, and the Sol System has an extensive Javelin defense system, jointly developed and operated by the superpowers.
​
Fortunately, there has never been an attempted Javelin strike.
​
RPCs / Quakes
A Javelin defense system like that in the Sol System would be far too expensive for Aurora, Hongzin, and IMEC. But the colonies have an advantage over Earth: their orbits can be adjusted.
​
A Random Positional Change, as it’s called on Aurora, is when the colony fires its thrusters to protect against a potential Javelin strike. As the name suggests, RPCs are conducted at random, sometimes up to four times a day, sometimes as little as twice a week. Because a Javelin would be traveling so fast, by the time its radar could pick out the colony, it would be just a second or two away, not enough time to sufficiently adjust course. This means it would require a local agent of some sort to transmit position information.
​
While RPCs don’t eliminate the threat of Javelins, they make a potential strike more expensive, more complex, and less likely to succeed. This is achieved at little extra cost, as the colony has thrusters anyway to avoid large asteroids in irregular orbits.
​
When you’re in the colony during an RPC, it feels like the ground is shifting under your feet and like you’re being pushed gently in one direction or another. This is commonly called a quake. It can induce mild vertigo because you feel the world moving but it doesn’t look like it’s moving.
​
The colony sends a warning to people’s cuffs shortly before each RPC for safety (for example, if someone is on a ladder). An alert is also sent to all autonomous vehicles.